Diabetes in America is in the public domain of the United States. You may use the work without restriction in the United States.
NCBI Bookshelf. A service of the National Library of Medicine, National Institutes of Health.
Lawrence JM, Casagrande SS, Herman WH, et al., editors. Diabetes in America [Internet]. Bethesda (MD): National Institute of Diabetes and Digestive and Kidney Diseases (NIDDK); 2023-.
Summary
Persons with diabetes make up the largest single group of kidney failure patients requiring dialysis or transplant in the United States. The high count reflects the growth in diabetes prevalence and increased access to dialysis and transplantation. Kidney failure affects about 1% of persons with diabetes in the United States; a considerably higher proportion, about 40%, have less severe kidney disease. Based on data from the National Health and Nutrition Examination Surveys 2017–March 2020, 14% of adults age ≥20 years or an estimated 35.5 million individuals had chronic kidney disease (CKD), and 38.7% of adults with diabetes had CKD-defined single measurements of albuminuria and serum creatinine.
Improvements in the management of persons with diabetes and CKD have extended the time course from onset of severe albuminuria to kidney failure and reduced the occurrence of cardiovascular events. Newer antihyperglycemic treatment strategies with sodium-glucose cotransporter-2 (SGLT2) inhibitors, glucagon-like peptide-1 (GLP-1) receptor agonists, and the newer mineralocorticoid receptor antagonists (MRAs) have shown efficacy in slowing kidney disease progression and reducing cardiovascular outcomes, without increasing the risk of hypoglycemia. In addition, landmark studies have shown that persons with kidney disease associated with diabetes require multidisciplinary management involving a combination of treatments and behavioral adjustments to delay progression of CKD and to prevent the associated complications.
Introduction
Important progress has been made since Diabetes in America, 3rd edition, was published in understanding the course and determinants of diabetes-related kidney disease (DKD) and its treatment (1). A new equation was developed for estimating glomerular filtration rate (GFR) that does not include a race term (2,3). Our understanding of glomerular hemodynamics and how it affects DKD progression has evolved (4,5). The management of DKD in type 2 diabetes was revolutionized by new medication classes, including sodium-glucose cotransporter-2 (SGLT2) inhibitors and glucagon-like peptide-1 (GLP-1) receptor agonists (RAs), and newer mineralocorticoid receptor antagonists (MRAs), which are effective at slowing kidney disease progression in diabetes (6,7,8,9). Newly updated guidelines were published that considered these findings, as well as emerging data on the role of multidisciplinary approaches in the management of DKD (10).
Nevertheless, kidney disease remains a major cause of morbidity and mortality in persons with diabetes, as indicated by the dramatic increase in the number of persons receiving renal replacement therapy since the 1980s. Increased availability of dialysis and transplant and the rising prevalence of diabetes are primarily responsible for this trend. For 2022, approximately 25% of U.S. health expenditures, or $503.4 billion, were incurred by people with diabetes; 32% of the diabetes cost was attributable to associated chronic kidney disease (CKD) (11). Because kidney disease in diabetes is strongly associated with cardiovascular disease (CVD) and the development of kidney failure, the combined cost incurred by CKD and diabetes is associated with a greater percentage of the Medicare budget than that associated with congestive heart failure, another common and costly condition (12). People with diabetes and kidney disease represent 4.6% of the overall Medicare population and incur 7.5% of annual Medicare spending (12).
Projected increases in diabetes prevalence and the increasing frequency of both type 1 and type 2 diabetes in young people threaten to reverse the modest progress achieved with available treatments (13). Not only is the course of kidney disease in youth-onset type 2 diabetes more aggressive than in type 1 diabetes (14,15), but the Treatment Options for Type 2 Diabetes in Adolescents and Youth (TODAY) study suggests it may also be more treatment resistant than kidney disease in adults with type 2 diabetes (16). Although the development of new therapeutic options is essential for improving the management of this complex disease, diabetes prevention may ultimately offer the greatest benefit for stemming the rising tide of DKD.
This review has been updated to incorporate the substantial advances made in the past 6 years in understanding the pathogenesis, course, and management of CKD in persons with diabetes. It draws from recent publications, including large clinical trials and reflects the opinions of the authors.
Sources and Limitations of National Data on Kidney Disease and Diabetes
The National Health and Nutrition Examination Survey (NHANES) is a cross-sectional, national probability sample that has been conducted periodically since 1971 and continuously since 1999. NHANES data collected from individuals for the period 1988–2020 were used to assess CKD among a nationally representative U.S. sample. Participants self-reported diagnosed diabetes status, based on the question of “Other than during pregnancy, have you ever been told by a doctor or health professional that you have diabetes or sugar diabetes?” An advantage of the NHANES is that the survey includes a health exam in a mobile examination center where laboratory measures are collected. Thus, the NHANES has laboratory information on glycated hemoglobin (A1C) and fasting plasma glucose, so persons with undiagnosed diabetes can also be assessed. Participants self-reported demographic characteristics, CVD, and prescription medications. During the exam component, blood pressure was taken by a trained interviewer.
Statistical Methods
All estimates from national surveys are weighted to produce estimates that are nationally representative of the noninstitutionalized U.S. population. This article provides weighted standard errors for estimates in tables. The relative standard error (RSE = [SE/estimate]*100) is provided in tables and figures for estimates that are likely unreliable due to sample size. Estimates with RSEs >50% are censored.
Definition, Measurements, and Classification
Although CKD in people with diabetes is heterogeneous, clinically it is usually attributed to diabetes, unless biomarker or biopsy information indicates otherwise (10). In recognition of this limitation, this article will use the term diabetes-related CKD (DKD). Irrespective of etiology, CKD is defined by reduced kidney function or the presence of kidney damage for at least 3 months, regardless of kidney function (10). Kidney damage is ascertained by increased urinary markers, such as albuminuria, or by abnormal urinary sediment, abnormal imaging studies, or kidney biopsy (10). The clinical diagnosis of DKD is based largely on the finding of elevated excretion of urinary albumin in a person with diabetes in the absence of other kidney disease.
Persons are classified as having elevated albuminuria if the albumin-to-creatinine ratio (ACR) in at least two urine samples collected within 3–6 months is ≥30 mg/g in the absence of clinical or laboratory evidence of urinary tract infection (10). Elevated albuminuria, the earliest marker of diabetic kidney damage, is frequently associated with a progressive decline in kidney function and a high risk for kidney failure and CVD. Although albuminuria is a continuous risk factor, it is often arbitrarily divided into moderately increased albuminuria (ACR 30–299 mg/g)—generally characterized by stable kidney function and a greater risk for higher levels of albuminuria than an ACR <30 mg/g—and severely increased albuminuria (ACR ≥300 mg/g), associated with arterial hypertension and a high risk of kidney failure (Table 1) (10). Because albuminuria occurs as a continuum, the American Diabetes Association (ADA) recommends simply using the term albuminuria for an ACR ≥30 mg/g (17). Urinary albumin excretion can be determined from 24-hour, overnight, or shorter urine collection periods; however, urinary ACR measured in a first morning void specimen is highly correlated with the 24-hour albumin excretion rate (AER) and is therefore an established and recommended way to assess urinary albumin excretion. Differences in methods of urine collection, albumin and creatinine measurements, reporting of results, and lack of standardized reference intervals for ACR often make comparisons between studies difficult.

TABLE 1.
Albuminuria Categories According to KDIGO Classification
Reduced kidney function is defined by GFR <60 mL/min/1.73 m2, and kidney failure by GFR <15 mL/min/1.73 m2 (Table 2) (10). Accurate determination of GFR is best achieved by infusing special markers into the bloodstream that are filtered at the glomerulus, but not secreted or reabsorbed by the tubules (e.g., inulin, iothalamate, iohexol, or 51Cr-EDTA), and measuring their disappearance from the blood or their appearance in the urine. Because such testing is laborious and expensive to use in large populations, the National Kidney Foundation (NKF) now recommends using the new CKD-EPI creatinine equation refit without the race variable to calculate the estimated GFR (eGFR) (2,3). An equation based on serum cystatin C, an endogenous filtration marker that is less influenced than serum creatinine by variations in muscle mass, diet, and tubular secretion or an equation that includes both serum creatinine and serum cystatin C may also be useful first-line tests (2,3). Regardless of etiology, CKD is classified based on both albuminuria and eGFR categories. Figure 1 shows the risk stratification of these stages for mortality, kidney, and cardiovascular outcomes (10).

TABLE 2.
Kidney Function Categories According to KDIGO Classification
In persons with diabetes, CKD may or may not be caused by diabetes alone. In those with type 1 diabetes of ≥10 years duration, CKD should be attributed to diabetes in the absence of other documented causes of kidney disease (10). Among persons with type 2 diabetes in particular, an eye examination is a useful, simple, and noninvasive test for differentiating between CKD caused by diabetes or other causes (18,19). A meta-analysis of 2,012 pooled patients with diabetes and CKD indicated that diabetic retinopathy has a sensitivity of 0.65 (95% confidence interval [CI] 0.62–0.68) and specificity of 0.75 (95% CI 0.73–0.78) for CKD due to diabetes (18). The presence of diabetic retinopathy in those with severe albuminuria is strongly suggestive of diabetes as cause of CKD, whereas the absence of diabetic retinopathy in those with normal or moderate albuminuria and an eGFR <60 mL/min/1.73 m2 suggests DKD due to other causes (18,20). Clinical biopsy studies show that nondiabetic morphologic changes are present in 3%–69.5% of patients with DKD, depending on the population characteristics and clinical profile, as elaborated below.
Figure 1 illustrates the Kidney Disease: Improving Global Outcomes (KDIGO) CKD classification that reflects prognosis based on the combined measures of eGFR and albuminuria (10). The risk associations of GFR and albuminuria categories with renal, cardiovascular, and all-cause mortality outcomes are reviewed in the following sections. For screening and management of kidney disease in persons with diabetes, the NKF and ADA recommend annual ACR screening starting at 5 years duration of type 1 diabetes and at diagnosis of type 2 diabetes (10,17). Serum creatinine measurements and eGFR assessment are recommended at least annually in adults with diabetes, regardless of ACR level.
Pathophysiology and Clinical Course
DKD typically progresses through a number of phases in which albumin or protein excretion increases, and GFR may rise and subsequently fall, sometimes culminating in kidney failure (Figure 2) (21,22). A diagram of the clinical progression of DKD and some of the factors contributing to it is shown in Figure 3 (1,23,24,25,26,27,28,29). The clinical manifestations of kidney disease are similar in both type 1 and type 2 diabetes. The major histologic changes of DKD and their relationships with kidney disease progression are discussed in detail in the Morphometry section.
Albumin Excretion
Urinary albumin excretion is usually within normal limits at the diagnosis of type 1 diabetes, except when diabetic ketoacidosis is present, which may contribute to both glomerular and tubular injury (30,31). Moderate albuminuria in the early years of diabetes is associated with poor metabolic control but is frequently transitory (22,32,33,34,35,36) and rarely persistent in the first 5 years (37). With hypoglycemic and angiotensin-converting enzyme (ACE) inhibitor or angiotensin II receptor blocker (ARB) treatments, normalization of albuminuria occurs in 58% of persons with type 1 diabetes (38), whereas persistent regression without treatment is observed in 16% (39). A1C <8% (<64 mmol/mol), systolic blood pressure <115 mmHg, low levels of both total cholesterol (<198 mg/dL [<5.13 mmol/L]) and triglycerides (<145 mg/dL [<1.64 mmol/L]), and greater insulin sensitivity are associated with the regression of albuminuria (38,40,41). Persons with persistent moderate albuminuria often progress to severe albuminuria (36) over a period of 10–20 years (3%–4% per year) (39,42), with hypertension and proliferative retinopathy also developing with advancing disease. Once severe albuminuria develops, albuminuria regression is less frequent (36,43), and the eGFR generally falls at a variable rate (2–20 mL/min/year) (39). Other frequent causes of transitory albuminuria include prolonged orthostatic position, intense exercise, infections, and heart failure.
Because the onset of type 2 diabetes is more insidious and diagnosis is often delayed, poor glycemic control and elevated blood pressure may be present for several years before diagnosis, and therefore, albuminuria is frequently present at diabetes diagnosis, even in youth-onset type 2 diabetes (44). Approximately 3% of newly diagnosed persons with type 2 diabetes have severe albuminuria (45,46). The course of DKD in type 2 diabetes is more heterogeneous than in persons with type 1 diabetes, in part reflecting a greater heterogeneity of kidney lesions due to the relatively higher prevalence of nondiabetic kidney lesions, a consequence of the older age at diagnosis of type 2 diabetes and more frequent occurrence of other causes of CKD, such as hypertension and chronic nonsteroidal anti-inflammatory drug (NSAID) use (47,48). About 25% of persons with type 2 diabetes have moderate albuminuria after 10 years, and 50% of those who develop moderate albuminuria do so within 20 years of diagnosis (39). Compared with adult-onset type 2 diabetes, youth-onset type 2 diabetes is associated with a more aggressive phenotype of DKD. Indeed, 55% of young persons with type 2 diabetes develop moderate albuminuria within approximately 15 years of diagnosis (44).
Glomerular Hemodynamic Function
In healthy adults, the eGFR ranges from 90 to 140 mL/min/1.73 m2, is stable through mid-adult life, and declines by approximately 1 mL/min/year after age 50 years, with the onset of global kidney sclerosis (49,50,51,52). The onset of diabetes is associated with hemodynamic changes in the kidney circulation that lead to increased renal plasma flow, glomerular capillary hyperperfusion, and an increased glomerular transcapillary hydraulic pressure gradient (Figures 4 and 5) (53,54,55,56,57,58,59).
Glomerular capillary hypertension and the ensuing increase in filtration pressure are partly responsible for the elevation of the eGFR, but various other glomerular and tubular factors also influence the magnitude of the hyperfiltration (60,61,62). The prevalence of hyperfiltration, generally defined as an eGFR of at least two standard deviations above the age- and sex-specific mean eGFR in persons with normal glucose tolerance, varies from 40% to 60% in persons with type 1 diabetes and from 7% to 73% in those with type 2 diabetes (26,63,64,65,66,67). The large variations in these estimates are attributed to differences in age, race and ethnicity, body size, glycemic control (with hyperglycemia increasing glycosuria and hyperfiltration), duration of diabetes, absence of diet standardization, and methodologies used to measure and report kidney function among different populations. In addition, simple single compartment models of plasma disappearance curves will overestimate GFR, and other methods are needed to accurately measure GFR and define its normal range. Accordingly, the definition of whole-kidney hyperfiltration is arbitrary, since whole-kidney eGFR is a product of single nephron filtration and nephron mass, and the latter varies significantly among people (68). More recently, filtration fractions or peak GFRs are explored in longitudinal studies to better ascertain hyperfiltration.
Several investigators have reported a relationship between hyperfiltration and the subsequent development of moderate albuminuria and progressive DKD (69,70,71), but others have not (24,25,72). Hyperfiltration may also reflect a generalized vascular dysfunction related to diabetes that in turn predisposes to DKD (73,74). Innovative analyses have revealed distinct molecular pathomechanisms contributing to the development of hyperfiltration, such as putative ligand-receptor pairs and downstream intracellular targets linked to cellular crosstalk between endothelial and mesangial cells (10).
After the initial elevation at onset of diabetes, the eGFR decreases to a near-normal range in response to metabolic control in both type 1 and type 2 diabetes (75,76,77,78) but usually not to levels found in persons without diabetes (55,78,79,80). In some, this reversal could reflect the initiation of progressive kidney disease, as suggested by the appearance of global glomerular sclerosis and the fall in single-nephron filtration coefficient (81). In others, it could represent a purely functional change in kidney hemodynamics associated with improvement in diabetes control or simply the intrinsic variability in the eGFR in the absence of significant histopathologic changes (82). Distinguishing between these two potential causes of eGFR decline requires either observation of the eGFR over a long period of time to determine whether it plateaus, reverses direction, or continues to decline to pathologic levels, or attention to other biomarkers, including albuminuria.
Coincident with the initial elevation of the eGFR at the diagnosis of diabetes is a slightly elevated urinary albumin excretion, although levels in the moderate albuminuria range or above are usually seen only after several years of diabetes (83). The eGFR in persons with moderate albuminuria is higher on average than in those with normal urinary albumin excretion (84,85). In those with severe albuminuria, it is lower, although in persons with type 2 diabetes, the eGFR may still be within the normal range (86,87). Cross-sectional data suggest that eGFR declines in persons with severe albuminuria, reflecting progressive glomerulosclerosis and loss of filtration surface area. Longitudinal studies confirm this hypothesis (88,89). The absence of albuminuria, however, does not preclude the presence of progressive kidney damage (see the Incidence of Elevated Albuminuria section) (90).
Kidney hemodynamic alterations induced by hyperglycemia and hypertension are believed to cause mechanical stretch and shear stress on endothelial cells and the mesangium, activating complex biochemical pathways that increase extracellular matrix production, hyperglycemia-induced injury, and podocyte damage and loss. These alterations ultimately lead to defects in selective glomerular capillary permeability, albuminuria, protein extravasation into the glomerular mesangium, expansion of mesangial matrix, and glomerulosclerosis (91,92,93,94,95,96,97,98). Trajectories of specific intraglomerular parameters estimated with the Gomez equation (99,100), namely the balance in vascular resistance between the afferent and efferent arterioles and the intraglomerular pressure, each predict incident kidney failure after adjusting for kidney function and both parameters correlate with mesangial fractional volume, a structural predictor of progressive DKD (4,101). Integration of long-term eGFR trajectories with morphometric and molecular analyses of research kidney biopsies identified an endothelial stress response with concomitant activation of downstream mesangial cell pathways associated with hyperfiltration providing a potential link between hyperfiltration and molecular pathways in the kidneys that lead to structural injury and progressive DKD (5).
Morphometry
The histologic changes in the kidneys of persons with type 1 diabetes and CKD are well described, typically homogenous, and predict development of elevated albuminuria, kidney failure, and cardiovascular death (102,103,104). On the other hand, the kidney lesions underlying CKD in type 2 diabetes are more heterogeneous (105,106). Longitudinal studies suggest a relationship between kidney lesions and eGFR decline (107,108). This section reviews the morphometric characteristics of DKD.
To appear in the urine, albumin must cross each layer of the glomerular filtration barrier consisting of the endothelial surface layer, fenestrated endothelial cells, glomerular basement membrane, and the glomerular epithelial cells or podocytes. Elevated albuminuria is an early clinical indicator of the destruction of the endothelial surface layer at the glomerular level (109,110,111,112), reduction in size and density of endothelial cell fenestrations (113,114,115), and thickening of the basement membranes. Subsequent expansion of the mesangium, consisting of mesangial cells and mesangial matrix, and podocyte injury and detachment further increase albuminuria, decreasing the available capillary filtration surface and leading to glomerulosclerosis. In addition to the glomerular morphologic lesions, diabetes progressively affects the kidney tubules, interstitium, and arterioles.
Glomerular basement membrane thickening strongly associates with albuminuria and eGFR impairment, suggesting that it is a good marker of early DKD. This alteration is followed by an increase in the mesangial volume per glomerulus (fractional mesangial volume), which is correlated inversely with eGFR, and positively with albuminuria and hypertension (65,116,117), and is therefore a strong predictor of progressive kidney dysfunction (Figure 6) (118). Virtually all persons with type 1 diabetes and advanced kidney disease have markedly thickened glomerular basement membrane and diffuse mesangial expansion. About 25% of persons with >10 years of diabetes duration present with Kimmelstiel-Wilson nodules, which are rounded, paucicellular, lamellated accumulations of mesangial matrix at the periphery of the glomerulus (119,120). Kimmelstiel-Wilson lesions associate with longer diabetes duration, higher serum creatinine, and more severe diabetic retinopathy (121). Mesangial expansion changes the architecture of the glomerular tuft, restricts the cellular component, distorts and occludes glomerular capillaries, decreasing the available capillary filtration surface and contributing to the decline in kidney function. Glomerular hypertrophy may compensate for the loss of filtration surface area, providing a means by which the eGFR is maintained in progressive kidney disease (122).
Podocyte injury appears to play an essential role in the progression of DKD. The podocyte, with its extended foot processes, provides structural support for the glomerular capillaries, as well as hydraulic resistance, and prevents the escape of proteins into the urinary space (123). With glomerular hypertrophy, the podocytes, which have a limited proliferative potential (124,125,126,127), stretch their foot processes more broadly to maintain coverage of the expanded glomerular basement membrane, a compensatory mechanism believed to influence their functional integrity (128). In addition, glomerular endothelial cell dysfunction, which precedes podocyte injury (121), appears to contribute to the latter through several mechanisms, including protein overload and toxicity at the podocyte level due to saturation of clearance mechanisms (129), increased shear stress (110), decreased endothelial nitric oxide synthase (eNOS) expression and activity (130,131), and production of cytokines, proteoglycans, and growth factors (113). Podocytes are known to absorb excess albumin arriving in the surrounding urinary space, and this increased workload may initiate inflammatory signaling and contribute to changes in podocyte-associated molecules and foot process effacement (132,133). Sustained mechanical stress and glomerular hypertension may ultimately lead to podocyte detachment and loss in the urine, leaving areas of bare glomerular basement membrane that further enhance loss of protein (Figure 7) (115). These denuded areas may initiate glomerular-tubular junction abnormalities and focal or global glomerular sclerosis (134). In persons with either type 1 (135) or type 2 (115,136) diabetes, podocyte changes may occur even before the increase in ACR, suggesting that diabetes itself may induce podocyte alterations. In a study of persons with type 2 diabetes, moderate albuminuria was associated with 20% and severe albuminuria with 40% podocyte loss relative to normal albuminuria (128). Moreover, individuals with moderate albuminuria had a 35% decline in the number of podocytes per glomerulus, and one-half of them progressed to severe albuminuria during 4 years of follow-up (81). Similarly, a lower number of podocytes and changes in the shape of the remaining podocytes were found in persons with type 2 diabetes and elevated albuminuria when compared with those with normal levels of albuminuria and similar fractional mesangial volume, indicating that changes in podocyte structure and density occur early during DKD and contribute to albuminuria. Other cross-sectional and experimental studies have reported similar findings (126,137). Some data suggest a repair mechanism via recruitment of parietal epithelial cells that is overwhelmed when podocyte loss exceeds a modest threshold (138,139,140). Consequently, significant damage to the podocytes is a potential starting point for irreversible glomerular injury in DKD.
In contrast with mesangial expansion, expansion of the interstitium, i.e., the intertubular, extraglomerular, extravascular space of the kidneys, primarily involves a cellular component represented by T lymphocytes and macrophages that infiltrate the interstitium, with subsequent fibrosis and declining kidney function (141,142). Efferent and afferent arteriolar hyalinosis, consisting of intramural accumulations of plasma proteins and lipids within kidney arterioles, may occur within a few years of diabetes onset (116,143,144). Although afferent arteriolar hyalinosis is a less specific diabetic lesion, both lesions are associated with elevated albuminuria and progression of kidney disease. Similar exudative lesions may occur in the glomerular capillaries (hyalinosis), Bowman’s capsule (capsular drops) (145,146), or proximal convoluted tubules and are generally associated with advanced DKD (116).
Advanced DKD is characterized by a marked reduction in the number of functioning glomeruli and further compensatory enlargement of those that remain functional. This stage is associated with a markedly reduced eGFR.
The intra- and extraglomerular morphologic changes described in this section progress at variable rates. Nonetheless, in one study, glomerular structural lesions explained 95% of the variability in albuminuria and 78% of eGFR variability (118,147). The latter percentage increased to 92% by adding indices of glomerular-tubular junction abnormalities and interstitial expansion to the regression models (147).
Albuminuria, however, is neither a sensitive nor specific early biomarker of progression to kidney failure, and the absence of albuminuria does not exclude the presence of relatively advanced diabetic kidney lesions and progressive kidney damage (148,149,150,151,152,153,154,155,156). New biomarkers of DKD appear to have additional prognostic information beyond that provided by albuminuria. These markers include kidney injury molecule 1 (KIM-1), liver fatty acid-binding protein (L-FABP), N-acetyl-β-D-glucosaminidase (NAG), neutrophil gelatinase-associated lipocalin (NGAL) (157,158,159,160,161,162,163,164,165,166,167,168,169,170,171,172,173), β-trace protein, β2-microglobulin (174,175,176), and tumor necrosis factor receptors 1 and 2 (177,178,179,180,181,182,183,184). Tumor necrosis factor-alpha receptor 1 and receptor 2 consistently enhance the discrimination of the survival models for kidney failure beyond that achievable by the clinically recognized risk factors in persons with type 1 or type 2 diabetes (177,178,179,180,181,182,183,184). Evaluation of other biomarkers in relation to DKD often shows conflicting results (157,158,159,160,161,162,163,164,165,166,167,168,169,170,171,172,173). This may be due to differences in study design, inclusion of persons without diabetes, use of surrogate or composite outcomes, or incomplete covariate adjustment in risk models (173). Nevertheless, combinations of biomarkers may enhance our ability to predict progressive DKD. A signature of 17 circulating inflammatory proteins enriched in tumor necrosis factor-receptor superfamily members, for example, was strongly associated with the 10-year risk of kidney failure in both type 1 and type 2 diabetes and in those with early and advanced stages of DKD (185).
Selective Glomerular Permeability
The glomerular capillary wall serves as a filter that discriminates among molecules on the basis of size, electrical charge, and configuration. Studies of glomerular filtrate collected by micropuncture or narrow size fractioning of exogenous polymers, such as dextran, indicate that albuminuria is primarily the result of impairment of the electrostatic barrier within the glomerulus, consequent to a decrease in endothelial cell glycocalyx (186,187) and heparan sulfate content of the glomerular basement membrane (188,189), and by changes in size selectivity across the glomerular capillary wall (Figure 8) (64,190,191,192,193,194,195,196,197,198,199,200,201,202,203,204,205,206).
A comparison of the mean dextran sieving profiles in 43 Pima Indians with type 2 diabetes and initially moderate albuminuria, who were followed for up to 8 years, showed no difference in the size selectivity of the glomerular filtration barrier between participants with moderate albuminuria and participants with long-term normal albuminuria (207). However, participants with severe albuminuria after 4 years of follow-up had a significantly higher fractional clearance of the large-radius test molecules than normoalbuminuric controls, with a reduction at the low-radius end, as shown in Figure 9. When the macromolecular shunt was analyzed as a function of albuminuria, an abrupt transition was apparent at an ACR of approximately 3,000 mg/g (Figure 10), whereas the contribution of the shunt in the moderate albuminuria range was very modest. These data suggest that permselectivity defects in the glomerular filtration barrier have little or no role in the development of moderate albuminuria. By contrast, a primary contributor to severe albuminuria is the shunt resulting from the presence of large pores within the glomerular capillary wall through which plasma proteins can easily pass (208,209,210,211). Morphometric data in participants with severe albuminuria identified a significant correlation between the shunt magnitude and podocyte foot process width (Figure 11) (207), which was not discernible in participants with moderate albuminuria. A similar size-selectivity defect has been reported in type 1 diabetes (203,208). These findings are consistent with the view that permselectivity defects responsible for increased albumin excretion may be focal and likely due to podocyte foot process effacement and simplification and, possibly, to defective intercellular junctions (212).
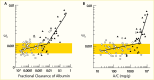
FIGURE 10.
Graph of the Shunt Magnitude as a Function of Albuminuria. The shunt magnitude parameter (ω0) as a function of albuminuria, as reflected by (Panel A) the fractional clearance of albumin and (Panel B) the urinary albumin-to-creatinine ratio (A/C), (more...)
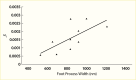
FIGURE 11.
Relationship Between Shunt Magnitude and Mean Podocyte Foot Process Width. Shunt magnitude (ω0) and mean foot process width in 10 persons with severe albuminuria who had kidney biopsies.
Subsequent studies using high-quality imaging techniques confirm that glomerular filtration barrier permeability to macromolecules is largely restricted to areas of podocyte damage (213,214,215).
Elevated Albuminuria
As noted earlier in this review, urinary albumin excretion is often increased at diagnosis of both types of diabetes but frequently returns to normal with the institution of glycemic control (22,32,33,34). Persistent albuminuria at the onset of type 2 diabetes, however, may reflect diabetes that has remained undiagnosed for years (34) or the presence of kidney disease unrelated to diabetes, since other kidney diseases are common at the ages when type 2 diabetes typically develops. On the other hand, elevated albuminuria is found in persons with impaired glucose tolerance (216,217,218), raising the possibility that hyperglycemia, even at levels below those diagnostic of diabetes, is sometimes associated with kidney abnormalities. Treatment with ACE inhibitors or ARBs at the highest tolerated dose is recommended in individuals with albuminuria, diabetes, and hypertension to slow decline in kidney function. The addition of SGLT2 inhibitors is also recommended in patients with type 2 diabetes, DKD, and an eGFR ≥20 mL/min/1.73 m2. MRAs in addition to ACE/ARBs and SGLT2 inhibitors further reduce albuminuria and kidney disease progression, as discussed in the Treatment of Diabetes-Related Kidney Disease section of this review (10).
Prevalence of Elevated Albuminuria
The prevalence of elevated albuminuria defined as ACR ≥30 mg/g in the U.S. adult population age ≥20 years with self-reported diabetes was 35.5%, 32.7%, 30.4%, 27.9%, and 31.5% based on one-time random spot urine measurements collected for the NHANES 1988–1994, 1999–2004, 2005–2010, 2011–2016, and 2017–2020, respectively (Table 3). When measured in a first morning void sample, the prevalence of ACR ≥30 mg/g in NHANES 2009–2010 participants with self-reported diabetes was 15.7% (95% CI 12.3%–19.0%) (219), prevalence of moderate albuminuria (ACR 30–299 mg/g) was 11.9% (95% CI 9.7%–14.4%), and the prevalence of severe albuminuria (ACR ≥300 mg/g) was 3.8% (95% CI 2.2%–6.4%). These estimates were significantly lower than the 24.1% prevalence (95% CI 19.8%–28.2%) of ACR ≥30 mg/g obtained from a random spot urine in the same population (prevalence of moderate albuminuria 19.3%, 95% CI 16.0%–23.1%, and severe albuminuria 4.8%, 95% CI 3.1%–7.4%). Prevalence of elevated ACR was significantly lower in the NHANES 2009–2010 population without diabetes (6.2%, 95% CI 4.9%–7.5%, in random urine samples; 3.7%, 95% CI 2.9%–4.5%, in first morning voids) (219). As mentioned in the Definition, Measurements, and Classification section, ACR measurement in first morning urine is a more reliable indicator of albumin excretion, since it correlates better with the 24-hour AER than the ACR measured in random spot urine. This finding suggests that ACR measurements based on single random spot urine specimens—the standard measurement in the NHANES—likely overestimate the frequency of elevated ACR in the general population (220,221,222).

TABLE 3.
Prevalence of Chronic Kidney Disease (eGFR and ACR) in Adults Age ≥20 Years, by Age, Sex, Race and Ethnicity, and Risk Factor Categories, U.S., 1988–2020
Because of the high intraindividual variation of ACR, both NKF and ADA guidelines (10,17) recommend confirmation of elevated albuminuria in a repeat measurement—either first morning void or random urine sample. The prevalence of persistent albuminuria, defined as elevated ACR in two consecutive random urine measurements within 2 weeks, was 15.9% in adults with diabetes (defined by A1C ≥6.5% [≥48 mmol/mol] or use of glucose-lowering medicines) in the NHANES 2009–2014, lower than the 20.8% prevalence in the NHANES III (1988–1994) (age, sex, and race and ethnicity adjusted prevalence ratio 0.76, 95% CI 0.65–0.89) (223). This overall change was due to lower albuminuria prevalence in adults age <65 years and non-Hispanic White adults over time. By contrast, the prevalence of a low eGFR increased during the same time in the overall population with diabetes. Updated trends in albuminuria and an eGFR <60 mL/min/1.73 m2 in the general U.S. population with diabetes are shown by age group in Table 4 and by race and ethnicity in Table 5. Based on the race-agnostic eGFR, the prevalence of a low eGFR in the U.S. population with diabetes has increased over time among non-Hispanic White adults. Compared with non-Hispanic White adults, the prevalence of a low eGFR was higher among non-Hispanic Black adults and lower among Mexican American adults but remained level in these two populations during the same time period (Table 5).

TABLE 4.
Prevalence of Albuminuria and Decreased Glomerular Filtration Rate in Adults Age ≥20 Years With Diabetes, by Age and Time Period, U.S., 1988–2020

TABLE 5.
Prevalence of Albuminuria and Decreased Glomerular Filtration Rate in Adults Age ≥20 Years With Diabetes, by Race and Ethnicity and Time Period, U.S., 1988–2020
By design, the NHANES does not differentiate the type of diabetes, and results in the adult population with self-reported diabetes largely reflect the experience of persons with type 2 diabetes. In a new analysis of NHANES 2011–2020 data conducted for Diabetes in America, the prevalence of DKD by type of diabetes was estimated using a published algorithm (224) to define persons with type 1 diabetes (Table 6). Results showed a higher prevalence of DKD in those with type 2 diabetes than in those with type 1 diabetes, regardless of sex or race and ethnicity. For both type 1 and type 2 diabetes, DKD prevalence was higher with age and was higher in non-Hispanic Black than in non-Hispanic White persons. DKD was also more frequent in persons with hypertension or CVD, regardless of type of diabetes.

TABLE 6.
Crude Prevalence of Chronic Kidney Disease in Adults Age ≥20 Years, by Type of Diabetes, U.S., 2011–2020
A cross-sectional, clinic-based study of 24,151 patients from 33 countries worldwide with type 2 diabetes, mean diabetes duration of 8 years, and without previously known albuminuria found overall prevalences of moderate and severe albuminuria of 39% and 10%, respectively (225). Compared with White patients, who had the lowest prevalence of albuminuria, Asian and Hispanic patients had nearly twice the odds of albuminuria (adjusted odds ratios [OR] 1.8, 95% CI 1.59–1.97, and OR 1.7, 95% CI 1.47–1.94, respectively). African patients were younger and had shorter known duration of diabetes than other racial and ethnic groups, but the odds of albuminuria were 1.5-fold higher than in White patients (95% CI 1.20–1.83), who had the lowest A1C levels and the highest frequency of antihypertensive, lipid-lowering, and anticoagulant or antiplatelet medication usage. Among Pima Indians with type 2 diabetes (217), 26% had moderate albuminuria and 21% had severe albuminuria, and in the population on the Western Pacific island of Nauru (218), 41% had moderate albuminuria and 31% had severe albuminuria. In an observational cohort in Japan, 25% had moderate albuminuria and 5% had severe albuminuria (226), while among primary care patients with type 2 diabetes in Singapore, 14.2% had moderate albuminuria and 5.7% had severe albuminuria (227). Figure 12 shows the prevalence of moderate and severe albuminuria in Pima Indians according to duration of diabetes (217). Although different methods and definitions of albuminuria were employed in these studies, other factors must be invoked to explain the large differences in the prevalence of elevated albuminuria in these different groups, including access to care, delays in the diagnosis of diabetes, differences in blood pressure and metabolic control, diet, and perhaps genetic susceptibility to DKD.
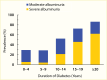
FIGURE 12.
Prevalence of Elevated Urinary Albumin Excretion in Pima Indians, by Duration of Diabetes, 1982–1988. Moderate albuminuria is defined as 31–299 mg/24 hours and severe albuminuria as ≥300 mg/24 hours.
Incidence of Elevated Albuminuria
Moderate albuminuria predicts the development of severe albuminuria in persons with type 1 (228,229,230,231,232) or type 2 (233,234,235,236) diabetes. In persons with type 1 diabetes, persistent albuminuria rarely develops in the first 10 years after diagnosis (237,238,239). The rate of progression to severe albuminuria is highest between 10 and 20 years duration of diabetes, and subsequently, the incidence rate declines (142,240,241). In the Diabetes Control and Complications Trial (DCCT), the cumulative incidence of persistent moderate albuminuria was 14%, 33%, and 38% at 10-, 20-, and 30-years duration of diabetes, respectively, among persons assigned to conventional treatment (mean A1C 9.6% [81 mmol/mol] at the time of incident albuminuria), which was higher than among those in the intensive treatment arm (10%, 21%, 25%, respectively; mean A1C 8.9% [74 mmol/mol] at the time of incident albuminuria). In 325 participants with incident moderate albuminuria who were followed for up to 23 years, the 10-year cumulative incidence was 28% for severe albuminuria, 15% for impaired eGFR (<60 mL/min/1.73 m2 at two consecutive study visits), and 4% for kidney failure (initiation of dialysis or kidney transplant) (35). The 10-year cumulative incidence of regression from persistent microalbuminuria to normoalbuminuria was also common, at 40%. Lower levels of A1C, blood pressure, low-density lipoprotein (LDL) cholesterol, and triglycerides and absence of retinopathy were associated with reduced risk of kidney disease progression.
In persons with type 2 diabetes, the incidence of albuminuria in relation to diabetes duration is more difficult to characterize because of the uncertainty in dating the true onset of diabetes in most studies. No relationship between duration of type 2 diabetes and the incidence of proteinuria was found in the Mayo Clinic population in Rochester, Minnesota (242), whereas in Wisconsin (243), a relationship between diabetes duration and incidence of proteinuria was stronger in persons who received insulin than in those who did not. In Pima Indians, in whom the duration of type 2 diabetes is known with greater accuracy because of systematic periodic oral glucose tolerance testing in the population, the age-sex-adjusted incidence of proteinuria, defined as urinary protein-to-creatinine ratio ≥0.5 g/g, was strongly related to duration of diabetes (244).
A secular decline in the incidence of DKD has been described in type 1 diabetes (245,246,247,248,249,250,251). In the Pittsburgh Epidemiology of Diabetes Complications (EDC) study (247), the cumulative incidence of DKD after 20 years of diabetes, defined as persistent AER >200 µg/min in timed urine collections, was 37% lower in the 179 participants diagnosed with diabetes in 1975–1980 than in the 339 participants diagnosed in 1965–1974. Among those with >25 years of diabetes duration, the declining trend was not statistically significant; Figure 13 shows a comparison of the EDC study (247) with data from the Steno study in Denmark (246). Nevertheless, significant reductions in both mortality and kidney failure rates in this population suggest a slower progression to kidney failure with improved management of diabetes complications. Comparable data have been reported for persons with type 1 diabetes in Sweden (248), where the cumulative incidence of persistent albuminuria (≥1 positive test by Albustix) after 20 years of diabetes decreased from 28% in persons diagnosed with type 1 diabetes in 1961–1965 to 6% in those diagnosed in 1971–1975. Furthermore, none of the 51 persons in whom type 1 diabetes was diagnosed in 1976–1980 developed persistent albuminuria during 12–16 years of follow-up. Figure 14 shows the cumulative incidence of persistent albuminuria in these cohorts according to the calendar year of diagnosis of diabetes. The decline in the cumulative incidence coincided with improvement in glycemic control comparable to that in the intensively treated group in the DCCT study (252). These findings were replicated in the Steno Diabetes Center cohort from Denmark (249,250). The cumulative incidence of DKD, defined as persistent albuminuria, after 20 years of diabetes duration declined from 31.1% in those with onset of type 1 diabetes in 1965–1969 to 13.7% in those with onset of diabetes in 1979–1984, with the most significant decline occurring in the most recent cohort (Figure 15) (250). This change paralleled a significant trend for earlier initiation of antihypertensive treatment following the onset of diabetes, increase in renin-angiotensin-aldosterone system (RAAS) inhibitor usage, and sustained improvement in mean blood pressure.
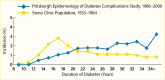
FIGURE 13.
Incidence of Kidney Disease in Persons With Type 1 Diabetes, Pittsburgh Epidemiology of Diabetes Complications Study, 1986–2000, and Steno Clinic Population, 1933–1984. Kidney disease is defined as an albumin excretion rate >200 µg/min (more...)
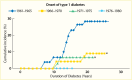
FIGURE 14.
Cumulative Incidence of Persistent Albuminuria in Persons With Type 1 Diabetes Diagnosed Before Age 15 Years, by Duration of Diabetes. Persistent albuminuria is defined as ≥1 positive test by Albustix. Subjects are divided into four groups based (more...)
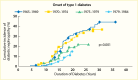
FIGURE 15.
Cumulative Incidence of Diabetic Nephropathy by Period of Onset of Type 1 Diabetes Diagnosed Before Age 15 Years, by Duration of Diabetes.
In contrast with type 1 diabetes, no secular decline in the incidence of proteinuria has been reported in type 2 diabetes. The 10-year cumulative incidence of persistent proteinuria in the predominantly Caucasian population age ≥40 years of Rochester, Minnesota, remained 12% in those diagnosed with type 2 diabetes in 1970–1979 (n=483) and those diagnosed in 1980–1989 (n=680) (253). The 20-year cumulative incidence of proteinuria reported in this study, however, was 41%, higher than the 25% cumulative incidence reported in an earlier Rochester study of individuals diagnosed with diabetes in 1945–1969 (242). These secular differences may be related in part to differences in age distributions and in diabetes diagnosis criteria between the studies. In the Pima Indian longitudinal study, in which the population was screened approximately every 2 years using 2-hour oral glucose tolerance testing, the incidence of proteinuria rose between 1967 and 2002 in response to the longer average duration of diabetes in this population in recent years (Table 7) (254).

TABLE 7.
Incidence of Proteinuria in Three Independent Time Periods Among Pima Indians With Type 2 Diabetes, by Diabetes Duration and Proportion of Person-Years Accumulating in Short and Long Duration Categories, 1967–2002
The progression of kidney disease in persons with newly diagnosed type 2 diabetes in the United Kingdom Prospective Diabetes Study (UKPDS) is presented in Figure 16 (255,256). The rate of progression to the next level of kidney disease severity (moderate albuminuria, severe albuminuria, or elevated plasma creatinine or renal replacement therapy) was 2%–2.8% per year; the risk of death grew with increasing severity of kidney disease, with an annual rate of 1.4% for subjects with no nephropathy, 3.0% for those with moderate albuminuria, 4.6% for those with severe albuminuria, and 19.2% with elevated plasma creatinine or renal replacement therapy. Individuals with severe albuminuria were more likely to die in any year than to develop kidney failure. Progression from no nephropathy to severe albuminuria or more advanced kidney disease was low (0.1%), as was progression from moderate albuminuria to elevated plasma creatinine or renal replacement therapy.
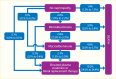
FIGURE 16.
Chronic Kidney Disease Progression in Persons With New-Onset Type 2 Diabetes, United Kingdom Prospective Diabetes Study, 1977–1997. Percentages represent annual rates with 95% confidence intervals.
Although moderate albuminuria was associated historically with an inexorable progression to severe albuminuria and kidney failure (257,258), a substantial proportion of persons with type 1 or type 2 diabetes and moderate albuminuria spontaneously regress to normoalbuminuria. This observation suggests that moderate albuminuria represents reversible kidney injury rather than the onset of an inevitable progression to kidney failure. On the other hand, other causes of transitory elevation in albuminuria need to be considered and excluded, such as orthostatic (postural) proteinuria, intercurrent illness, or exercise. In a prospective observational study of persons with type 1 diabetes, baseline diabetes duration of about 18 years, and moderate albuminuria, only 19% developed severe albuminuria, whereas 59% regressed to normal albuminuria after 6 years of follow-up (38). The incidence of albuminuria reported in predominantly White populations with type 1 diabetes is lower and the rate of regression higher than those reported in Black persons with type 1 diabetes for a similar follow-up time (259,260). In 473 Black persons with type 1 diabetes and baseline mean diabetes duration of 10.4 years, the 6-year cumulative incidence of moderate and severe albuminuria was 26.0% (95% CI 20.9%–31.6%) and 16.9% (95% CI 12.6%–21.8%), respectively, with an overall incidence of elevated albuminuria of 42.9% (95% CI 36.9%–50.0%). Incidence of any proteinuria in this population was positively associated with diabetes duration at baseline in men, whereas in women, it declined after 10 years duration of diabetes (Figure 17) (259). Among the 370 Black persons with moderate albuminuria at baseline, 23.5% (95% CI 19.3%–28.2%) progressed to severe albuminuria. Overall, 33.5% progressed to a worse albuminuria category, and 8.5% improved either spontaneously or due to treatment with ACE inhibitors or other antihypertensive medicines.
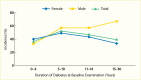
FIGURE 17.
Six-Year Incidence of Any Proteinuria in African American Men and Women With Type 1 Diabetes, by Duration of Diabetes at the Baseline Examination, New Jersey 725 Study, 1993–1998.
In general, the proportion of persons with type 2 diabetes who regress from moderately increased albuminuria to normoalbuminuria is 30%–54%, while the frequency of progression to overt proteinuria is 12%–36% (261,262,263). Moderate albuminuria of short duration, use of RAAS inhibitors, lower A1C (<6.9% [<52 mmol/mol]), and systolic blood pressure <129 mmHg are independently associated with albuminuria regression. Among 750 American Indians from Arizona, Oklahoma, and North and South Dakota age 45–74 years in the Strong Heart Study, albuminuria was measured in 1989–1991 (baseline), 1993–1995 (second examination), and 1997–1999 (third examination) (264). Among those with normal ACR at baseline, 67% remained so and 33% developed elevated albuminuria (29% moderate albuminuria and 4% severe albuminuria) by the second examination. More participants with normal albuminuria at the second examination remained normoalbuminuric (77%), and 23% developed elevated albuminuria (19% moderate albuminuria and 4% severe albuminuria) by the third examination, suggesting a decline in onset of kidney disease in the later years. Risk factors for onset of elevated albuminuria were higher A1C, higher systolic blood pressure, higher baseline ACR, smoking, glucose-lowering treatment, and longer diabetes duration. Participants with a baseline ACR between 10 and 30 mg/g had nearly threefold higher risk of elevated ACR over a 4-year period (OR 2.7, 95% CI 1.9–3.9) than those with baseline ACR <5 mg/g (264). The cumulative incidence of elevated ACR increased with duration of diabetes (p<0.01) and was similar in men and women in the three study centers (Figure 18). Although elevated albuminuria generally precedes the decline in kidney function, in youth-onset type 1 diabetes and in adults with type 2 diabetes, the latter may occur without evidence of albuminuria (265,266). However, this phenomenon has not been described in youth-onset type 2 diabetes. A higher prevalence of albuminuria and more rapid progression to kidney disease in youth-onset type 2 diabetes compared with youth-onset type 1 diabetes have been reported (265,266).
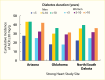
FIGURE 18.
Four-Year Cumulative Incidence of Elevated Albuminuria in American Indians, by Duration of Diabetes, Sex, and Study Site, Strong Heart Study. ACR, albumin-to-creatinine ratio.
While elevated albuminuria occurs earlier during type 2 than type 1 diabetes, sustained control of A1C and systolic blood pressure associate with higher likelihood of regression to lower or normal levels regardless of type of diabetes. To date, secular declines in the incidence of albuminuria are reported in type 1 diabetes only. Some studies in persons with diabetes report declines in the eGFR without preceding albuminuria; however, whether lack of albuminuria was due to RAAS treatments or infrequent follow-up remains unclear.
Diabetes-Related Kidney Disease as a Risk Factor for Kidney Failure, Cardiovascular Disease, and Death
A new analysis of the NHANES 1999–2018 conducted for Diabetes in America shows cardiovascular mortality, cancer-related mortality, and all-cause mortality among persons with DKD, defined by albuminuria or low GFR (Table 8). For albuminuria, these associations are a continuum, starting at levels well below the 30 mg/g threshold that defines moderate albuminuria (267,268,269,270). Among American Indians age 45–74 years in the Strong Heart Study (267) who were free of CVD at baseline, high-normal ACR level was a strong and independent predictor of all cardiovascular events, including nonfatal and fatal CVD, and these associations were similar in individuals with or without diabetes. In those with diabetes, the adjusted risks for all, nonfatal, and fatal CVD events increased by 20% (hazard ratio [HR] 1.20, 95% CI 1.07–1.34), 13% (HR 1.13, 95% CI 0.99–1.28), and 48% (HR 1.48, 95% CI 1.17–1.87), respectively, for each doubling of ACR within the normal range.

TABLE 8.
Crude All-Cause and Cause-Specific Death Rates in Adults With Diabetes and Chronic Kidney Disease, U.S., 1999–2018
Among persons with diabetes participating in the NHANES III, those with the highest ACR levels (≥300 mg/g) and lowest eGFR (15–59 mL/min/1.73 m2) had a 2.7-fold higher risk for cardiovascular mortality and a 2.5-fold higher risk for all-cause mortality, relative to those with normal ACR and an eGFR ≥90 mL/min/1.73 m2 (271). Similar associations were found in participants without diabetes; however, those with diabetes had greater absolute risks for these outcomes. Adjustments for diabetes duration and cardiovascular risk factors did not change the significance of these associations, suggesting that CKD is a risk amplifier, with much of the excess CVD in diabetes occurring in persons with DKD.
Nearly all of the excess mortality associated with either type of diabetes is found in persons with severe albuminuria (37,272,273), primarily from kidney disease or CVD in persons with type 1 diabetes (272,274), from CVD in White persons with type 2 diabetes (275), and from CVD or kidney disease in Pima Indians with type 2 diabetes (273,276). Among individuals with type 1 diabetes in the EDC study, those who maintained normal levels of albumin excretion had the lowest standardized mortality ratio at 10- and 20-year follow-up, and they were more likely to die from nondiabetes-related causes than individuals who had elevated albuminuria (AER ≥20 μg/min) (277). Other studies in persons with more than 30 years duration of type 1 diabetes show similar associations (278,279).
An analysis of secular trends in the incidence of kidney failure and mortality in patients with type 1 diabetes and severe albuminuria from the Joslin Clinic, however, found no significant decline in pre-kidney failure death rate, progression to kidney failure, or post-kidney failure death rate from 1991 to 2004, despite both widespread adoption of kidney protective treatments during the same period and significant improvements in blood pressure and total serum cholesterol concentration (280,281). Sixty-nine percent of either pre- or post-kidney failure excess mortality was attributable to CVD. Moderate and severe albuminuria independently predicted all-cause mortality in a monotonic fashion in the Finnish Diabetic Nephropathy study (FinnDiane) cohort, which represented 16% of the population with type 1 diabetes in Finland (282). During 7 years of follow-up, the overall death rate in persons with moderate albuminuria was nearly three times that observed in the general Finnish population (adjusted standardized mortality ratio [SMR] 2.8, 95% CI 2.0–4.2), with CVD representing 56% of these deaths. Severe albuminuria was associated with nine times the death rate observed in the age- and sex-matched general population (adjusted SMR 9.2, 95% CI 8.1–10.5), of which 45% was caused by CVD. Indeed, the impact of albuminuria level on mortality risk was equivalent to that of preexisting macrovascular disease, as defined by a history of myocardial infarction, unstable angina requiring hospitalization, coronary revascularization, stroke, carotid surgery, peripheral revascularization, or amputation for critical limb ischemia. Other major causes of death included infections and cancer. By contrast, overall death rates in persons with normal ACR were equivalent to those in the general population (adjusted SMR 0.8, 95% CI 0.5–1.1), regardless of diabetes duration, indicating that DKD is a major driver of excess mortality in type 1 diabetes.
Irrespective of albuminuria level, the eGFR was independently associated with mortality, but in a U-shaped fashion, as shown in Figure 19 (282), possibly reflecting confounding from other morbid conditions unrelated to kidney disease at high eGFR levels, such as serum creatinine confounding due to reduced muscle mass, increased tubular secretion, and extrarenal elimination of creatinine. Moreover, measurement imprecision is greater at lower concentrations of serum creatinine, compounding the difficulty of interpreting serum creatinine levels in those with a normal or high-normal eGFR (283).
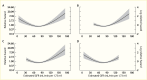
FIGURE 19.
Risk of Death Associated With Estimated Glomerular Filtration Rate in Type 1 Diabetes. Relative hazard computed in persons with type 1 diabetes: (Panel A) without end-stage renal disease, (Panel B) with normoalbuminuria, (Panel C) with moderate albuminuria, (more...)
In type 2 diabetes, death rates due to both overall and cardiovascular causes are greatly increased with advancing kidney disease (284,285,286,287). Among 1,993 Pima Indians (55.9% with type 2 diabetes, the only type of diabetes occurring in this population, even at young ages) followed for a median of 11 years, death rates from natural causes increased with worsening kidney function in persons without or with diabetes (Figure 20) (276). Death rates in persons without kidney disease were virtually identical without or with diabetes and increased similarly with worsening kidney disease in both groups, suggesting that kidney disease rather than diabetes per se is the major determinant of increased mortality among the population with diabetes. The higher overall mortality in those with longer duration of diabetes is due primarily to the greater proportion of person-years of follow-up falling in the categories of worse kidney function (Figure 20C). The presence of kidney disease was associated with excess mortality from DKD, CVD, infections, and malignancy in the Pima Indians with diabetes and from infections in those without diabetes. Among 4,081 American Indians, 45% with diabetes, a reduced eGFR (<90 mL/min/1.73 m2) was associated with increased risk of CVD events, including coronary heart disease, stroke, and heart failure during a median follow-up of 15 years (288). The associations between eGFR measure and CVD events were attenuated after adjusting for albuminuria.
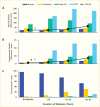
FIGURE 20.
Trends in Age-Sex-Adjusted Death Rates From Natural Causes and Cardiovascular Disease in American Indians With and Without Diabetes, by Severity of Kidney Disease. Trends in age- and sex-adjusted death rates from (Panel A) natural causes and (Panel B) (more...)
Fewer studies have focused on the noncardiovascular mortality associated with DKD. Regardless of the presence of diabetes, persons with kidney disease are three to four times more likely to have a poor prognosis after acquiring infections than those without kidney disease (289,290,291). Persons with diabetes have a higher risk of community-acquired lower respiratory tract infection, pneumonia, and sepsis with a declining eGFR and increasing ACR, independent of age, sex, smoking status, and comorbid conditions of diabetes. Although some reports suggest that kidney disease, particularly kidney failure, increases the risk of death from several types of cancer, e.g., bladder and kidney cancers (292,293,294), this is not a uniform finding (292,295,296,297).
Quantitative information about ACR adds significant predictive value to the eGFR about the risk of death or kidney failure, and therefore, using both ACR and the eGFR is significantly better for predicting these outcomes than using either measure alone (298,299,300). An analysis conducted in 10,640 persons with type 2 diabetes enrolled in the Action in Diabetes and Vascular Disease: Preterax and Diamicron Modified Release Controlled Evaluation (ADVANCE) trial showed similar contributions of high ACR and a low eGFR to cardiovascular and kidney events (Figure 21) (299). These findings concur with those from a systematic review of the association between microvascular and macrovascular disease in type 2 diabetes (301), which showed an approximately twofold increased risk for cardiovascular events associated with albuminuria or a reduced eGFR (Table 9) (268,301,302,303,304,305,306,307,308,309,310,311,312,313,314). The strength of these associations remained after adjustments for multiple confounders, suggesting that microvascular and macrovascular disease in type 2 diabetes may share similar pathophysiologic mechanisms. A meta-analysis of 1,024,977 participants (nearly 13% with diabetes) from 30 general population and high-risk cardiovascular cohorts and 13 CKD cohorts indicated that while the absolute risks for all-cause and cardiovascular mortality are higher in the presence of diabetes, the relative risks of kidney failure or death by eGFR and ACR are similar with or without diabetes (315). These findings underscore the importance of kidney disease per se as a predictor of important clinical outcomes, regardless of the underlying cause of kidney disease.
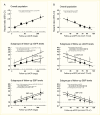
FIGURE 21.
Association of (A) Urinary Albumin-to-Creatinine Ratio and (B) Estimated Glomerular Filtration Rate Levels During Follow-Up With the Risk for Cardiovascular Events. Closed and open squares represent hazard ratios (HRs) in subgroups for eGFR <60 (more...)

TABLE 9.
Prospective Studies With at Least 200 Type 2 Diabetes Subjects That Evaluated Hard Cardiovascular Endpoints
Kidney Failure
Prevalence of Diabetes-Related Kidney Failure (End-Stage Kidney Disease)
Kidney failure, or stage 5 CKD, is the most advanced stage of CKD, requiring renal replacement therapy, such as dialysis or kidney transplant, for survival. Since 1988, all persons receiving treatment for kidney failure, regardless of age and insurance coverage, are included in the United States Renal Data System (USRDS) registry (12). Consequently, the annual data report published by the USRDS contains the most complete and reliable information on treated kidney failure in the United States.
Table 10 summarizes the incidence and prevalence of treated kidney failure according to demographic characteristics, primary diagnosis, and treatment modality in the United States in 2020 (12). In that year, 130,522 new cases of dialysis and transplant were added to the national registry, of which 59,474 (46%) had kidney disease attributed to diabetes.

TABLE 10.
Summary Statistics on Reported Kidney Failure Treatment, by Age, Sex, Race and Ethnicity, and Primary Diagnosis, U.S. Renal Data System, 2020
Diabetes and hypertension represent the leading causes of kidney failure in the United States (Figure 22) (12). In 1985, the adjusted prevalence of treated kidney failure attributed to diabetes was 103 cases per million population; these patients accounted for 19% of prevalent treated kidney failure in the United States. By 2020, the prevalence had risen to 859.7 cases per million population, representing 38% of prevalent treated kidney failure in the United States (45.5% of dialysis patients and 21.6% of kidney transplant patients) (Table 10) (12). An increasing prevalence of diabetes and improved CVD survival are responsible, in part, for this growth (Figure 23) (316). About 30% of persons with type 1 diabetes and 10%–40% of those with type 2 diabetes eventually develop kidney failure. Because type 2 is the predominant type of diabetes, it also far exceeds type 1 diabetes as a cause of diabetes-related kidney failure. Of 309,017 prevalent cases of treated kidney failure with diabetes at the end of 2020, type 2 diabetes was responsible for 269,980 (87%; Table 11) (12).
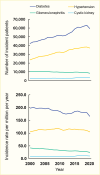
FIGURE 22.
Trends in Incident End-Stage Kidney Disease Cases and Incident Rate, by Primary Diagnosis of End-Stage Kidney Disease, U.S. Renal Data System, 2000–2020. Data are adjusted for age, sex, and race and ethnicity; unknown sex and other or unknown (more...)
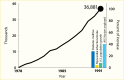
FIGURE 23.
Incident ESKD Cases (Line) and Increase in ESKD Attributable to Increased Prevalence of Diabetes, Improved Survival Following Myocardial Infarction and Stroke, and U.S. Population Growth in 1991 (Bars). ESKD, end-stage kidney disease.

TABLE 11.
Prevalence of Reported End-Stage Kidney Disease, by Primary Diagnosis, Sex, and Race and Ethnicity, U.S. Renal Data System, 2020
Incidence of Diabetes-Related Treated Kidney Failure
In 1985, the adjusted incidence of kidney failure attributable to diabetes, treated with renal replacement, was 45 cases per million population. The rate increased to 170 per million by 2005 and leveled off thereafter (12). The increasing prevalence of diabetes and more inclusive criteria for initiating renal replacement therapy contributed to higher incidence rates of diabetes-related kidney failure over time.
Trends in the incidence of treated kidney failure due to diabetes differ broadly by age and race and ethnicity (Table 12). The adjusted incidence of treated kidney failure decreased between 2000 and 2020 by 7%, 24%, and 30% in White, Black, and Hispanic persons, respectively, but remains threefold and twofold higher in Black and Hispanic, respectively, compared with White persons with diabetes (12). Among American Indian/Alaska Native populations, incidence of treated kidney failure due to diabetes declined by 53% between 2000 and 2016, more than for any other racial and ethnic group, while it remained relatively stable in the Asian population (317). A study of 452,238 commercially insured and Medicare Advantage patients with CKD found that compared with White patients, Asian, Black, and Hispanic patients were more likely to receive guideline-recommended care in 2012–2019, including treatment with an ACE inhibitor, ARB, or statin, referral to a nephrologist, albuminuria monitoring, and long-term prescription NSAID avoidance, but were similarly or less likely to achieve blood pressure and diabetes control for slowing CKD progression (318). Racial differences in the incidence of treated kidney failure in persons with type 2 diabetes are also attributable in part to differences in the duration of diabetes (319,320,321,322,323,324,325,326). A shift towards a younger age at onset of type 2 diabetes among some minority populations may be partly responsible for the secular trends in kidney failure incidence observed in the younger groups, as illustrated by studies in Pima Indians (327,328). Whereas the incidence of diabetic kidney failure in Pima Indians age ≥45 years declined after 1990, those age <45 years experienced no such decline. The lack of decline in the younger Pima Indians was associated with a lower percentage of RAAS inhibitor usage than in older subjects. Women of childbearing age were least likely to receive RAAS inhibitors, presumably because of concerns about their use in pregnancy.

TABLE 12.
Incidence Rates of Reported End-Stage Kidney Disease Due to Diabetes, by Age, Sex, and Race and Ethnicity, U.S. Renal Data System, 2000–2020
Epidemiologic data on racial and ethnic differences in the incidence of treated kidney failure in type 1 diabetes are sparse, in part because type 1 diabetes is less frequent, particularly among minority populations, and in part due to uncertainties related to diagnosis; young persons or those who are treated with insulin are often misclassified as having type 1 diabetes. According to USRDS data, of all new cases of treated kidney failure due to diabetes between 2016 and 2020, 91% were attributable to type 2 diabetes (Table 13) (12).

TABLE 13.
Incidence of Reported End-Stage Kidney Disease, by Primary Diagnosis, Sex, and Race and Ethnicity, U.S. Renal Data System, 2016–2020
Survival of Persons With Treated Diabetes-Related Kidney Failure
Persons with a primary diagnosis of diabetes treated for kidney failure have lower survival relative to other causes of kidney failure (12), primarily due to CVD (329,330,331,332), which continues to advance during the course of renal replacement therapy. While survival on dialysis has slowly improved across modalities since 2000 (Figure 24), it remains reduced in persons with diabetes, more than half of whom die within 5 years of beginning dialysis in the United States (Figure 25) (12). Among incident treated kidney failure patients with a primary diagnosis of type 1 diabetes in 2017–2021, 14.6% died during the first year, while the proportion of those receiving a kidney transplant in the first year was 6% (12).
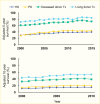
FIGURE 24.
Survival Probabilities Among Incident End-Stage Kidney Disease Patients With Diabetes, by Treatment Modality, U.S. Renal Data System, 2000–2015. Data are adjusted for age, sex, race and ethnicity, and primary cause of end-stage kidney disease. (more...)
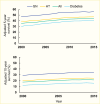
FIGURE 25.
Survival Probabilities Among Incident Dialysis Patients, by Cause of End-Stage Kidney Disease, U.S. Renal Data System, 2000–2015. Data are adjusted for age, sex, race and ethnicity, and primary cause of end-stage kidney disease. GN, glomerulonephritis; (more...)
Black (333,334), Hispanic (335), Asian (12), and American Indian (12,336) patients treated for diabetes-related kidney failure generally have a lower adjusted risk of death compared with White patients. In 2020, White patients with diabetes had the lowest expected remaining years of life on dialysis, and Asian patients had the highest expected survival (Figure 26) (12). Data from the USRDS also indicate that Asian patients with kidney transplant due to diabetes had the longest survival, whereas American Indians had the lowest number of expected remaining years of life. Among patients on hemodialysis due to DKD, the risk of death was 31% lower in American Indian, Black, or Hispanic patients and 38% lower in Asian patients compared with White patients. In 1995–2010, among American Indians, those with 100% Indian ancestry had the lowest adjusted risk of death compared with White patients (HR 0.58, 95% CI 0.55–0.61) (Figure 27) (337). The risk of death increased to 0.95 (95% CI 0.82–1.11) in those with less than one-quarter American Indian ancestry (337), suggesting that hereditary factors play a role in how patients respond to dialysis treatment. Kidney transplant recipients with diabetes have much better survival than those on dialysis, as shown in Figure 24 (12).
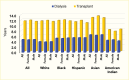
FIGURE 26.
Expected Remaining Years of Life Among Prevalent Dialysis Patients With Diabetes, U.S. Renal Data System, 2020. SOURCE: Reference (12)
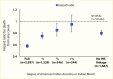
FIGURE 27.
Hazard Ratios for Death From Any Cause Among Non-Hispanic American Indians With Diabetes at Initiation of Hemodialysis, by Degree of American Indian Ancestry, 1995–2010. Information on American Indian ancestry was obtained from the IHS patient database. (more...)
Risk Factors for Diabetes-Related Kidney Disease
Numerous risk factors have been identified for the development and progression of DKD. In this section, the evidence for some of the more prominent factors is reviewed.
Duration of Diabetes
One of the most important risk factors for DKD is the duration of diabetes—its influence being far greater than that of age, sex, or type of diabetes. For a given duration of diabetes, the cumulative incidences of severe albuminuria and kidney failure are similar for type 1 and type 2 diabetes (315,320,338,339,340).
Sex-Related Differences
Sexual dimorphism may play a role in the risk of DKD, due to differences in multiple biologic processes that may differentially impact each sex. Differences in pathophysiology relating to sex hormones, kidney hemodynamic function, adiponectin concentrations, and oxidative stress may influence risk of DKD, although studies have shown heterogeneous results (341), possibly due to differences in methods used to measure kidney function and residual confounding. Nevertheless, the collective evidence suggests the greatest risk of DKD may be among postmenopausal women, followed by men and premenopausal women.
Socioeconomic Factors
Socioeconomic factors are often taken into consideration when describing associations between risk factors and DKD in large populations with diabetes. A low socioeconomic status, based on such factors as education, income, and occupation, is associated with increased prevalence of diabetes, hypertension, and CKD (342,343,344). In a study among older adults in the United States, experiencing financial hardship was associated with an average 50% increased risk of DKD compared with no financial hardship (345). Other socioeconomic factors that may affect DKD risk include environmental factors, such as poor access to nutrition, reduced green spaces, and air and water pollution (346). In a study of primary care patients in Pennsylvania with newly diagnosed diabetes, the highest quartile of community socioeconomic deprivation (based on area-level estimates of unemployment, educational attainment, poverty level, utilization of public assistance, and household vehicle access) was associated with approximately 30% higher odds of an eGFR <60 mL/min/1.73 m2 compared with the lowest quartile of deprivation (347). A systematic review reported that a majority of longitudinal studies found fine particulate matter (PM2.5) to be associated with increased risk of kidney disease, with diabetes as a potential exacerbator of the effects of PM2.5 on kidney function (348). Health literacy and access to healthcare and health insurance may also affect the development and progression of DKD (349). Exposure to an adverse prenatal environment, such as that caused by poor maternal dietary habits, smoking, or poor health, may also introduce adverse health traits that persist in subsequent generations (350).
Hyperglycemia
Increased blood glucose concentration is a major risk factor for the development and progression of moderate albuminuria in both types of diabetes (106,237,241,242,243,244,338,351,352,353,354,355,356,357,358,359,360,361,362,363) but may have a lesser influence on progression of more advanced kidney dysfunction (353,364), when hypertension, hypercholesterolemia, and genetic factors play a greater role in shaping the outcome (38,352). In the EDC study, participants with type 1 diabetes and A1C >10% (>86 mmol/mol) had a 3.6-fold higher risk of developing moderate albuminuria than those with lower A1C levels. Glycemic control was the only predictor of moderate albuminuria in both men and women, regardless of diabetes duration (352). Higher 2-hour post-load plasma glucose concentration (Figure 28), fasting plasma glucose, and A1C in Pima Indians with type 2 diabetes were associated with a higher incidence of elevated ACR after adjustment for age, sex, and duration of diabetes (244,354). Similarly, among American Indians age 45–74 years with type 2 diabetes from Arizona, Oklahoma, and North and South Dakota, higher fasting plasma glucose and A1C among those with normal baseline ACR and serum creatinine were associated with increased risk of elevated albuminuria (264).
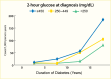
FIGURE 28.
Incidence of Proteinuria in Pima Indians With Type 2 Diabetes, by OGTT Glucose Level and Diabetes Duration. Incidence of proteinuria (protein-to-creatinine ratio ≥1.0 g/g) by duration of diabetes in 480 Pima Indians with type 2 diabetes, according (more...)
Hyperglycemia induces hyperfiltration, a predictor of progressive kidney disease (67,69,70,365,366). In humans, therapeutic interventions that improve glycemic control reduce hyperfiltration in both type 1 and type 2 diabetes (96,367).
Evidence for the role of hyperglycemia in the development of diabetic glomerular lesions comes from biopsy studies in identical twins discordant for type 1 diabetes (368) and from morphologic studies before and after pancreas transplantation (369). Glomerular changes, including widened glomerular and tubular basement membranes and increased mesangial fraction, were identified only in the diabetic member of twin pairs (Figure 29), suggesting that metabolic status, and not genetic predisposition, is responsible for the development of diabetic kidney lesions (368). Prolonged normoglycemia following pancreas transplant in persons with type 1 diabetes and established DKD promotes virtually complete reversal of glomerular and tubular basement membrane thickness and of increases in mesangial and interstitial volumes (Figure 30) (369).
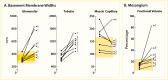
FIGURE 29.
Morphometric Measurements in Kidney and Skeletal Muscle From Identical Twins Discordant for Type 1 Diabetes. Panel A: basement membrane width (nm). Panel B: fractional volume of the mesangium (%). Values for twins without diabetes (●) are linked to (more...)
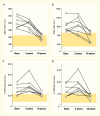
FIGURE 30.
Renal Histologic Changes at Baseline and 5 and 10 Years After Pancreas Transplantation. Thickness of the GBM (Panel A), thickness of the TBM (Panel B), mesangial fractional volume (Panel C), and mesangial matrix fractional volume (Panel D) are shown before (more...)
Hypertension
High blood pressure is related to DKD in many cross-sectional and longitudinal studies of both type 1 and type 2 diabetes. In type 1 diabetes, this relationship frequently reflects elevation of blood pressure in response to kidney disease (370,371,372,373). In type 2 diabetes, the onset of hypertension generally precedes DKD and is often associated with obesity. The risk of kidney disease is three times as high in persons with type 1 diabetes who have a hypertensive parent as in those whose parents are not hypertensive (Figure 31) (374). In addition, those with kidney disease have a higher prevalence of parental hypertension and a higher mean arterial blood pressure during adolescence (375). Increased blood pressure during sleep may herald the development of moderate albuminuria in adolescents and young adults with type 1 diabetes, as shown in Figure 32 (376). In that study, blood pressure was monitored at 2-year intervals for up to 9 years or as long as the ACR was in the normal range; a ratio of ≤0.9 between the mean nighttime systolic pressure and the mean daytime systolic pressure defined the dipping during sleep. Moderate albuminuria did not develop in those with poor metabolic control in whom blood pressure remained normal, suggesting that nocturnal elevation in blood pressure may identify those with type 1 diabetes who are most susceptible to progression of kidney disease. Prolonged hypertension may lead to kidney damage through multiple mechanisms (377), including hardening and narrowing of the renal arterioles with reduction in renal perfusion volume. Damage to the endothelium can reduce the synthesis of nitric oxide, leading to further vascular damage. Podocyte dysfunction may also ensue, due to maladaptive responses from cell injury and mechanical stress from increased intraglomerular pressure.
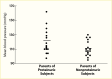
FIGURE 31.
Mean Blood Pressure in Parents of Persons With Type 1 Diabetes and With or Without Proteinuria. Family study of diabetes, including 26 surviving parents of 17 persons with type 1 diabetes and proteinuria and parents of 17 matched persons without diabetes (more...)
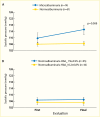
FIGURE 32.
Nocturnal Systolic Blood Pressure According to Albuminuria and Level of Glycated Hemoglobin (A1C) in Type 1 Diabetes. Panel A: Nocturnal systolic blood pressure in 14 subjects who subsequently developed moderate albuminuria (microalbuminuria) and in 61 subjects (more...)
In Pima Indian offspring with type 2 diabetes, the prevalence of proteinuria was similar if neither parent or only one parent had hypertension (8.9% and 9.4%, respectively) but was significantly higher if both parents had hypertension (18.8%), after adjustment for age, sex, duration of diabetes, and 2-hour post-load plasma glucose concentration in the offspring and diabetes in the parents (378). The odds for proteinuria in the offspring when both parents had hypertension was 2.2 times (95% CI 1.2–4.2) that when only one parent had hypertension. This relationship was present even when controlled for the effects of blood pressure and its treatment in the offspring. In addition, higher blood pressure before the onset of type 2 diabetes was related to a higher prevalence of elevated albuminuria after the onset of diabetes, suggesting that blood pressure plays a causal role in the development of DKD (Figure 33) (379).
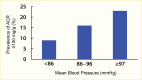
FIGURE 33.
Prevalence of Elevated Albuminuria in Pima Indians After Diagnosis of Type 2 Diabetes, by Blood Pressure Before Onset of Diabetes. Elevated albuminuria is defined by urinary albumin-to-creatinine (ACR) ratio ≥100 mg/g. Participants with the highest (more...)
Sodium-lithium countertransport activity, a genetically influenced trait, is often higher in persons with essential hypertension and in those whose parents have essential hypertension (380,381,382,383). In type 1 diabetes, elevated rates of countertransport activity are reported in persons with moderate albuminuria or proteinuria (Figure 34) (384,385,386,387), elevated GFR (388), and proliferative retinopathy (389). These findings suggest that persons with diabetes and hypertension and with elevated sodium-lithium countertransport activity are at greater risk for DKD and possibly other microvascular complications, although these findings have not been uniformly confirmed (387,390,391). Few studies assessed this relationship in those with type 2 diabetes; some found an association between higher sodium-lithium countertransport activity and albuminuria (392,393,394), and others did not (395,396).
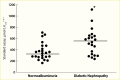
FIGURE 34.
Correlation of Sodium-Lithium Countertransport Activity With Nephropathy. Sodium-lithium (Na-Li) countertransport activity was measured in 21 persons with type 1 diabetes and normoalbuminuric matched controls. Diabetic nephropathy is defined by a urinary (more...)
Lipids
Many of the abnormalities in plasma lipoproteins associated with kidney disease are sequelae of kidney dysfunction, yet dyslipidemia may also play a role in the pathogenesis of glomerular injury (397,398,399). Persons with pre-dialysis DKD typically have significant hypertriglyceridemia, elevated LDL, and low high-density lipoprotein (HDL) cholesterol concentrations. These abnormalities are more pronounced in persons with severe albuminuria than those with moderate albuminuria (35,400,401,402) but tend to subside with progression to uremia and dialysis (Table 14) (403). Besides quantitative changes, lipid particles in people with diabetes change qualitatively; LDL and HDL particles tend to be smaller and denser with advancing CKD (402,404).

TABLE 14.
Changes in Lipids, Lipoproteins, ApoA, and ApoB, by Stage of Chronic Kidney Disease
In a study of persons with type 1 diabetes, LDL cholesterol predicted progression of DKD as defined by a doubling of albuminuria or a decline in creatinine clearance in excess of 3 mL/min/year (405). In that study, LDL cholesterol was associated with elevated albuminuria after nearly 9 years of follow-up; a higher triglyceride content of very low-density lipoprotein (VLDL) and intermediate-density lipoprotein (IDL) particles predicted worsening of moderate albuminuria, whereas smaller LDL size was associated with declining kidney function in persons with severe baseline albuminuria. These findings suggest that specific lipids or lipid profiles may influence the onset or progression of DKD, although the findings have not been consistently observed (352,406,407,408).
In type 2 diabetes, prospective studies with long follow-up found that LDL cholesterol increases the risk for severe albuminuria (409) and kidney failure (410). In a post hoc analysis of 1,061 persons with type 2 diabetes included in the Reduction of Endpoints in Non-insulin dependent diabetes with the Angiotensin II Antagonist Losartan (RENAAL) study, the risk of kidney failure was 32% higher for each 50 mg/dL (1.30 mmol/L) increase in LDL cholesterol concentration and 67% higher for each 100 mg/dL (2.59 mmol/L) increase in total cholesterol concentration; lowering LDL cholesterol concentrations with a statin reduced the 1-year risk of kidney failure, although concurrent treatment with losartan, an ARB, likely contributed to improving this outcome by ACR (410). Elevated plasma triglycerides are associated with increased risk for both moderate and severe albuminuria (409) and with kidney failure in type 2 diabetes (411). In addition, low concentrations of HDL cholesterol predict increased risk of albuminuria progression in persons with type 2 diabetes and moderate albuminuria (412,413). In the UKPDS, each mmol/L decline in HDL cholesterol was associated with nearly threefold risk of doubling of serum creatinine concentration (HR 2.78, 95% CI 1.01–7.68) 15 years after the diagnosis of type 2 diabetes (409). The Strong Heart Study examined the relationship between plasma lipoprotein concentrations and the risk of elevated albuminuria in 671 American Indians with type 2 diabetes who were followed for a mean of 3.9 years (414). A low HDL cholesterol concentration was associated with elevated ACR in women only (OR 0.56, 95% CI 0.32–0.98) after adjustment for age, duration of diabetes, hypoglycemic treatment, A1C, study site, degree of Indian ancestry, mean arterial blood pressure, baseline albumin excretion, insulin concentration, body mass index (BMI), alcohol consumption, and physical activity. The association between HDL cholesterol and increased ACR in women was largely explained by a HDL cholesterol concentration <0.9 mmol/L, with little additional effect at higher HDL concentrations (Figure 35) (414). No other lipids were found to increase the risk of incident albuminuria; high levels of total and VLDL triglycerides and small LDL size were positively, but not significantly, associated with abnormal albuminuria. In the absence of a standard measure of insulin resistance, however, the study could not determine whether low HDL cholesterol itself, insulin resistance, or other correlated variables were most prominently increasing the risk of nephropathy (414).
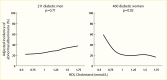
FIGURE 35.
Effect of Serum HDL Cholesterol Concentration on the Incidence of Albuminuria in American Indians With Type 2 Diabetes, Strong Heart Study, 1989–1996. Effect of HDL cholesterol concentration on the incidence of albuminuria (ACR ≥30 mg/g) (more...)
Dyslipidemia may contribute to onset and progression of DKD through mechanisms similar to those responsible for arterial atherogenesis (398,415,416). Hypercholesterolemia may impair the kidney’s hemodynamic responses and tubular function by decreasing nitric oxide production and/or increasing superoxide activity in the kidney (417,418,419,420,421), with resulting antidiuretic and antinatriuretic effects (422). Although not directly affecting GFR, these actions may play a role in the development of systemic hypertension associated with diabetes (422). Oxidized LDL and free fatty acids can cause structural and functional damage to podocytes by inducing mitochondrial dysfunction and accumulation of reactive oxygen species (423), suggesting a direct causal role in the development and progression of proteinuria. Experimental rodent models of type 1 and type 2 diabetes indicate that down-regulation of glomerular ATP-binding cassette transporter (ABCA1) expression can lead to excessive cholesterol accumulation in podocytes (424,425). Under normal conditions, ABCA1 mediates the efflux of cholesterol to lipid-poor apolipoproteins (primarily Apo A1) to form HDLs. This mechanism has also been demonstrated in glomerular transcripts from persons with DKD when compared with living normal controls (426,427). Nevertheless, a definitive role for dyslipidemia in the development and progression of DKD in humans remains to be established.
Dietary Protein
In epidemiologic studies, higher-protein diets are associated with insulin resistance and development of diabetes (428), as well as with greater risk of kidney damage or loss of function, especially in individuals with diabetes, hypertension, and/or reduced kidney function. These risks are mainly confined to animal meat intake. Vegetable or dairy proteins do not appear to adversely affect kidney health (429,430).
In experimental models, excessive protein intake causes kidney vasodilation and glomerular hyperperfusion and accelerates structural and functional injury in models of DKD, whereas low-protein diets offer kidney protection (431,432,433,434,435,436). Physiologic studies in humans confirm the hyperfiltration response found in animal studies, but only in the presence of chronic hyperglycemia (437,438). On the other hand, dietary protein of animal origin may be a significant source of advanced glycation endproducts, particularly when cooked at high temperatures or in fat (439). In vitro studies indicate that advanced glycation endproducts can produce kidney damage through a variety of mechanisms even from the very early stages of kidney disease, but their contribution to disease progression is still unclear.
Two other studies explored the association between CKD and dietary acid load, a surrogate for the intake of acid-inducing foods (rich in animal proteins) versus base-inducing foods (fruits and vegetables), quantified as the net acid excretion estimated from 24-hour dietary recall (440), and between CKD and metabolic acidosis (441). The former found that higher dietary acid load among 12,293 U.S. adult participants in the NHANES 1999–2004 was associated with an eGFR <60 mL/min/1.73 m2 or elevated albuminuria in participants with hypertension and in those without hypertension or diabetes. Older age, poverty, being Black or male, but not diabetes, were significantly associated with an increasing level of net acid excretion in this population. In the latter study (including 21% persons with diabetes), serum bicarbonate level ≤22 mEq/L was associated with a 54% higher risk of eGFR decline during a median follow-up of 3.4 years after adjustment for baseline eGFR and clinical, demographic, and socioeconomic patient characteristics. Since this study had no information on patients’ diet or medication during the follow-up, the findings cannot be attributed directly to dietary protein intake. Thus, although a theoretical case can be made for the impact of dietary protein on the development of DKD, no observational data in humans unequivocally support such a role.
Another study of NHANES data in adults found that a proinflammatory diet (high in cholesterol and saturated fats) and low intake of anti-inflammatory foods (fruits and vegetables) was associated with systemic inflammation and risk of kidney failure (442). Inflammation was estimated to mediate 36% of the effect of the proinflammatory dietary pattern on risk of kidney failure.
Smoking
A cross-sectional analysis of 61,675 participants in the NKF’s Kidney Early Evaluation Program (KEEP), 27.1% of whom had CKD, identified smoking along with obesity, diabetes, hypertension, and CVD as significant factors associated with CKD (443). Similarly, in 14,632 participants in the NHANES 1999–2004, 15.3% of whom had CKD, current smoking increased the odds of CKD by 31% (443).
In a large population-based study, maternal smoking during pregnancy increased the odds of albuminuria in the full-term offspring who later developed type 1 diabetes by threefold, independent of low birth weight or blood pressure levels (444). In young persons with type 1 diabetes who smoked, the risk of albuminuria was nearly threefold higher than in nonsmokers (445). Likewise, the frequency of proteinuria (>500 mg/24 hours) was twice as high in smokers as in nonsmokers of similar age, duration of type 1 diabetes, A1C, and prevalence of hypertension (446). On the other hand, no relationship was observed between smoking and GFR decline in a longitudinal study of persons with type 1 diabetes with albuminuria and serial 51Cr-EDTA GFR measurements over a median follow-up of 7 years (Figure 36) (447), although other smaller studies identified an association (448,449,450).
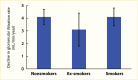
FIGURE 36.
Impact of Smoking Habit on Kidney Function, Adjusted for Difference in Blood Pressure Between Groups, Steno Clinic, 1983–1997. GFR was measured yearly by the 51Cr-EDTA plasma clearance technique. In 301 persons with type 1 diabetes and severe (more...)
In men with type 2 diabetes, cigarette smoking was associated with higher A1C and ACR levels in a dose-dependent fashion (451), with nearly one-half of the heavy smokers having A1C >9% (>75 mmol/mol) and ACR ≥30 mg/g. The odds of elevated ACR were 2.8–3.2 times as high in those smoking more than 15 pack-years than in nonsmokers, regardless of age, blood pressure control, or diabetes duration. This and other studies in type 2 diabetes suggest that smoking is associated with kidney damage regardless of blood pressure control and treatment with RAAS inhibitors (452,453). Smoking also increased the risk of moderate or severe albuminuria (adjusted OR 1.50, 95% CI 1.21–1.86) after 5 years of follow-up in a large population-based study of persons with type 2 diabetes and no kidney disease at baseline from the Swedish National Diabetes Registry, although no association was found with the rate of kidney function decline (454).
In addition to nicotine, mainstream cigarette combustion produces more than 4,000 compounds, including reactive oxygen species, carbon monoxide, nitric oxide, toxic metals, and polycyclic aromatic hydrocarbons, which may add to an already increased susceptibility to DKD (455). Although the precise mechanisms are unclear, tobacco smoking is known to cause vasoconstriction, impair platelet function and coagulation, and alter blood pressure (456,457). Given that persons with diabetes already have widespread vascular damage as a consequence of their diabetes (458), smoking may accelerate the process.
Obesity
Obesity is a major risk factor for diabetes, hypertension, and CVD, all of which increase the risk for kidney disease. It increasingly affects young people, particularly among Hispanic, Black, and American Indian populations (459), leading to an earlier onset of diabetes and its major complications, including kidney disease (460). In a simulation study using data from the NHANES, lifetime risk of any-stage kidney disease has been estimated at 32.5% for individuals with normal weight, 37.6% with overweight, and 41.0% with obesity (461). The longitudinal population-based study in the Pima Indians showed that between 1965 and 2003, BMI increased in all age groups, including children. During the same time period, the incidence of diabetes increased nearly sixfold among those age <15 years, without a similar trend in the older ages. These findings suggest that the increasing prevalence and degree of obesity in youth combined with a nearly fourfold increase in the frequency of exposure to diabetes in utero have shifted the onset of diabetes to younger ages (462). For any diabetes duration, participants with youth-onset type 2 diabetes had a lower risk of kidney failure (p=0.007) than those with older-onset diabetes (Figure 37). Because of the longer duration of diabetes by mid-life, however, the incidence of kidney failure was higher between ages 25 and 54 years in those with youth-onset diabetes than in those with adult-onset diabetes (p<0.001) (Figure 37) (327). Given the continued increase in obesity, the experience in the Pima Indians may be relevant to other populations at high risk for diabetes.
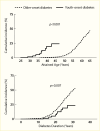
FIGURE 37.
Effect of Youth-Onset Type 2 Diabetes on Incidence of End-Stage Kidney Disease in Pima Indians, by Diabetes Duration and Age, 1965–2002. Participants were observed from onset of diabetes to outcome or December 2002. Youth-onset type 2 diabetes (more...)
Animal and human kidney biopsy studies describe obesity-associated adaptive increases in GFR and renal plasma flow, resulting from both proximal salt reabsorption and elevated angiotensin II, as well as structural changes, such as glomerular hypertrophy and focal segmental glomerulosclerosis (463). A retrospective clinical and histopathologic study of 6,818 native kidney biopsies found diabetes-like lesions in 45% of persons with obesity-related glomerulopathy (464). Further, the prevalence of obesity-related glomerulopathy, defined as glomerulomegaly with or without focal segmental glomerulosclerosis, increased tenfold over a 15-year period (from 0.2% in 1986–1990 to 2.0% in 1996–2000) (464). As the authors excluded other causes of focal segmental glomerulosclerosis, this increase was largely attributed to the rising prevalence of obesity in the general population. Notably, five individuals who lost significant weight experienced a 50%–75% decline in proteinuria, and their kidney function remained stable.
Periodontal Disease
Periodontal disease, which often occurs in the absence of diabetes, is a frequent complication of diabetes (465), contributing to poor glycemic control, low-grade chronic systemic inflammation, and increased risk of macrovascular and microvascular complications (465,466,467). Because diabetes is the leading cause of kidney failure, periodontitis is frequently present among dialysis patients, increasing the risk for cardiovascular death (466,468). Severity of periodontitis and being edentulous predicted severe albuminuria and kidney failure in a dose-dependent manner among adults with type 2 diabetes followed for a median of 9 years (469). A study investigating the relationships between diabetes, periodontal disease, and CKD in the NHANES 1988–1994 population suggested a bidirectional relationship between CKD and periodontal disease, with periodontitis associated with the risk of CKD both directly and mediated by hypertension and duration of diabetes, and CKD having a direct effect on periodontitis (470). Control of periodontal infection in adults with diabetes improves A1C level (465) and reduces the concentration of various markers of inflammation, coagulation, and adhesion (471,472). Whether such control also reduces the onset or progression of DKD is not known.
Other Risk Factors for DKD
Drug and Iodinated Contrast-Associated Nephrotoxicity
Cumulative toxicity from prolonged exposure to NSAIDs is a cause of CKD, particularly among the elderly and those with diabetes, underlying volume depletion, heart failure, or preexisting kidney dysfunction (473,474,475,476,477).
A variety of commonly used or prescribed medicines have been associated with tubulointerstitial and glomerular lesions, including hydralazine, levamisole, and antithyroid drugs (478). Two main mechanisms of interaction with kidney structures have been described: direct cellular toxicity and immune-mediated injury (479). These injuries and the related clinical parameters are generally reversible after withdrawing the offending drug. Nonetheless, establishing a causal link between drug exposure and the development of renal pathology is often difficult, due to lack of specific markers, recall bias, underlying diseases, heterogeneity of symptoms, and physicians’ unfamiliarity with the renal effects caused by certain drugs.
Iodinated contrast-induced nephropathy is one of the most commonly reported causes of acute kidney failure in hospitalized patients. Those with diabetes and an eGFR <60 mL/min/1.73 m2 are at particularly increased risk for contrast-induced nephropathy, especially with use of high-osmolar iodinated contrast media (480).
Autonomic Neuropathy
In the United States, between 25% and 28% of adults age ≥40 years with diabetes have peripheral neuropathy (481). Autonomic neuropathy with ensuing alteration of glomerular vascular resistance may hasten deterioration of kidney function in persons with type 1 diabetes (482). Whether autonomic neuropathy per se is part of the pathogenic process leading to DKD or is a reflection of the severity of diabetes is unclear (482,483,484). Nonetheless, the two microvascular complications of diabetes occur together frequently. One study reported that half of the deaths in those with type 1 diabetes and autonomic neuropathy were attributed to DKD (485).
Pregnancy
Among women with normal kidney function, regardless of the presence of diabetes, pregnancy is associated with a rise in the eGFR of about 50% that persists through the 37th week of gestation (283,486) and is accompanied by a moderate increase in urinary protein excretion (283,487). Table 15 summarizes ACR and kidney function characteristic of nondiabetic and diabetic pregnancies (488). Most women with DKD have successful pregnancy outcomes despite increased risk. Those with advanced kidney disease, poor glycemic control, or hypertension, however, are at increased risk of pregnancy complications and subsequent deterioration in kidney function (486).

TABLE 15.
Kidney Function During and After Pregnancy, by Presence or Absence of Diabetes
Although neither pregnancy nor parity adversely affects the course of early DKD (489), the few available studies suggest that women with more severe kidney impairment may be at greater risk of kidney disease progression later in life. In a retrospective review of pregnant women with type 1 diabetes, a creatinine clearance <90 mL/min or a urinary protein excretion >1g/day during the first 20 weeks of gestation was associated with a greater decline in kidney function at approximately 3 years after delivery than in those with less severe DKD during early pregnancy (490). On average, creatinine clearance declined from 120 mL/min in the first weeks of pregnancy to 78 mL/min at the 3-year follow-up (p=0.01), even as changes in proteinuria remained nonsignificant from the initial measurement during pregnancy (2.94±4.26 g/24 hours vs. 1.74±1.33 g/24 hours, p=0.25) (Table 16) (490). Mothers with advanced kidney disease were more likely to experience preeclampsia and have offspring with low birth weight. Worsening of preexisting DKD was also reported among women with type 1 diabetes who were followed for up to 26 years (491). In this study, morbidity and mortality remained higher in women with pregnancies 10 years after their last delivery than in those without pregnancies (491). Preeclampsia is more frequent in women with diabetes and is associated with 7.7-fold higher odds of subsequent DKD compared with those with normotensive pregnancies (492).

TABLE 16.
Serial Creatinine Clearance and 24-Hour Proteinuria Measurements During and After Pregnancy in Participants With Type 1 Diabetes, 1988–1994
Intrauterine Factors
Clinical studies indicate that exposure to a diabetic intrauterine environment increases the risk of kidney disease later in life, perhaps as a consequence of reduced nephron formation during fetal development (493,494,495). Individuals with reduced nephron endowment are also prone to develop hypertension and CVD. These conditions may be triggered or hastened by exposure to additional kidney insults, such as high-salt diet, obesity, and diabetes (493,494,496). Moreover, impaired nephrogenesis and hypertension may be passed to the next generation through changes in epigenetic gene regulation (497,498,499). Consistent quantitative information about this risk comes from longitudinal studies in Pima Indians. Exposure to diabetes in utero among Pima Indians increased nearly fourfold over a 30-year period, paralleled by a doubling in the prevalence of children with diabetes attributable to this exposure (500). Intrauterine exposure to diabetes was associated with a fourfold increase in the age-sex-adjusted incidence of kidney failure in young adults with type 2 diabetes, mediated largely by the younger age at onset of diabetes (Figure 38) (328). A study exploring the impact of intrauterine exposure to diabetes compared renal vascular resistance in 19 adult nondiabetic offspring of mothers with type 1 diabetes with 18 offspring of fathers with type 1 diabetes as control subjects (501). At baseline, exposed and control subjects had similar age (median 24 and 25 years, respectively), BMI, age at delivery, glucose concentrations, blood pressure, kidney size, and 51Cr-EDTA-measured GFR. Kidney vasodilatation induced with amino acid infusion was associated with significantly less increase in GFR and effective renal plasma flow and less decline in mean arterial pressure in offspring of mothers with type 1 diabetes, suggesting a reduced kidney functional reserve, possibly due to low nephron number and compensatory permanent single nephron hyperfiltration. A proposed explanation for these observations is that exposure to a diabetic intrauterine environment causes differential apoptosis during nephrogenesis via increased intrarenal renin-angiotensin system activation and nuclear factor (NF)-kappaB signaling (502,503).
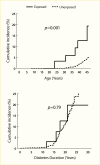
FIGURE 38.
Cumulative Incidence of Diabetic End-Stage Kidney Disease Among Pima Indians, by Offspring’s Age and Duration of Diabetes, According to Exposure to Diabetes in Utero, 1965–2006.
A critical shortage of maternal fuels during pregnancy may manifest as intrauterine growth retardation, defined as birth weight below the 10th percentile for gestational age. Low birth weight is frequent in minority populations, in populations undergoing rapid transition from traditional to modern lifestyle, and in those with low socioeconomic status, as well as in pregnancies associated with inadequate maternal weight gain, poor antenatal care, maternal hypertension, or smoking (504). In 12,364 adults with a history of diabetes or hypertension screened by the NKF’s KEEP, 15% of whom reported birth weight <2,500 g, a U-shaped relationship was found between birth weight and CKD among White men (505). Similarly, in a population-based, case-control study using 1987–2008 birth certificates from Washington state, low birth weight (defined as 400–2,499 g) was associated with a twofold higher risk of CKD in the offspring (OR 2.41, 95% CI 2.08–2.80) compared with those of normal birth weight (defined as 2,500–3,999 g); the higher odds persisted after adjustment for maternal diabetes, BMI, and smoking (OR 2.88, 95% CI 2.28–3.63) (Figure 39) (506). The same study found that children with CKD were more likely to be born to mothers with overweight or obesity (OR 1.25, 95% CI 1.10–1.41), regardless of maternal diabetes, gestational hypertension, and smoking (506).
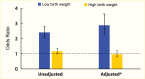
FIGURE 39.
Association Between Birth Weight and Chronic Kidney Disease. The horizontal dashed line represents the reference birth weight (defined as 2,500–3,999 g). Birth weight is based on Washington state birth records from 1987–2008. Chronic kidney (more...)
Familial and Genetic Factors
Familial aggregation of DKD and racial and ethnic differences in disease susceptibility suggest a genetic predisposition to DKD. Three initial studies reported familial clustering of DKD (507,508,509). In one study, DKD was reported in 83% (24 of 29) of the siblings of probands with type 1 diabetes and kidney disease but in only 17% (2 of 12) of the siblings of probands with type 1 diabetes without kidney disease (Figure 40) (507). Moreover, 41% of the affected siblings of persons with DKD had kidney failure. A similar study in persons with type 1 diabetes found DKD in 33% of the siblings of persons with DKD but in only 10% of the siblings of persons without DKD (508). Familial clustering is also found in type 2 diabetes. In two generations of Pima Indians with type 2 diabetes, the frequency of proteinuria in the offspring with diabetes was higher if both parents with diabetes had proteinuria than if neither did; if one parent had proteinuria, the prevalence was intermediate (Figure 41) (509).
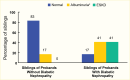
FIGURE 40.
Kidney Status of Siblings of Type 1 Diabetes Probands, by Diabetic Nephropathy. Probands with diabetic nephropathy were persons with kidney transplant for diabetic ESKD; probands without diabetic nephropathy were persons with albumin excretion rate <45 (more...)
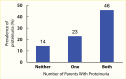
FIGURE 41.
Prevalence of Proteinuria in Offspring, by Number of Parents With Proteinuria, Pima Indians. Proteinuria is defined as urinary protein excretion ≥1g protein/24 hours. Data are adjusted for age, systolic blood pressure, diabetes duration, and glucose (more...)
A number of candidate genes have been identified that may be related to DKD. In Pima Indians, a potential susceptibility locus for kidney failure was found within the plasmacytoma variant 1 (PVT1) gene (510), which was confirmed in subjects of European descent with type 1 diabetes (511). Genome-wide association studies of subjects with type 1 diabetes in the Genetics of Kidneys in Diabetes (GoKinD) study (512) found single nucleotide polymorphisms (SNPs) in the FERM domain-containing protein 3 (FRMD3) gene and near the cysteinyl-tRNA synthetase (CARS) gene associated with DKD, defined by overt proteinuria or kidney failure. These associations were confirmed in the DCCT/Epidemiology of Diabetes Interventions and Complications (EDIC) prospective study of type 1 diabetes (513), and susceptibility loci near CARS were found to be common to both types of diabetes in another study (514). Engulfment and cell motility 1 (ELMO1) loci were associated with DKD in European (515) and Japanese (516) persons with type 1 diabetes and in Black persons with kidney failure due to type 2 diabetes (517). The Family Investigation of Nephropathy and Diabetes (FIND) study (518) collected DNA and cell lines from European American, Black, American Indian, and Hispanic American families in whom type 2 diabetes was the predominant cause of kidney disease. For all ethnicities combined, the strongest evidence for linkage to DKD was on the long arm of chromosomes 7, 14, 18, and on the short arm of chromosome 10; to ACR on the long arm of chromosomes 2, 7, and 15; and to the eGFR on the long arm of chromosomes 1, 7, and 8 (519,520).
A meta-analysis assessing the effects of all genetic variants associated with severe albuminuria or kidney failure found 24 reproducible genetic variants associated with DKD (Figure 42) (521). These genetic variants are involved in the renin-angiotensin system, polyol, oxidative stress, inflammation, angiogenesis, glomerular filtration barrier defects, cell growth, differentiation, and apoptosis pathways, supporting their roles in the pathogenesis of DKD (521). Another meta-analysis identified additional variants, primarily involved in kidney DNA methylation, that were associated with kidney disease (522). Albuminuria and, to a greater degree, GFR are heritable (523), but the actual genes responsible for DKD remain elusive. Although these findings are not universal, they do suggest that genetic factors may predispose some individuals to a higher risk of DKD than others.
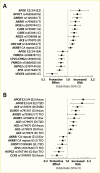
FIGURE 42.
Genetic Variants Reproducibly Associated With Diabetic Nephropathy. Panel A: All genetic variants in or near a gene that were reproduced in an independent study and significantly associated with diabetic nephropathy after meta-analysis. Panel B: All genetic (more...)
In addition to specific genetic factors, the multifaceted cross-talk between genes and environmental factors can induce tissue-specific epigenetic changes, i.e., heritable changes in gene expression without alterations in the DNA sequence that can lead to aberrant gene regulation expressed as pathologic phenotype. Epigenetic changes include DNA cytosine methylation, histone posttranslational modifications in chromatin, and noncoding RNAs—all of which can modulate diabetes complications through alterations in gene expression (Figure 43) (524). Through epigenetic mechanisms, for example, cells acquire metabolic memory of prior hyperglycemic exposure that appears to mediate the development and progression of DKD (525,526). Hyperglycemia-induced epigenetic aberrations alter transcription factors involved in the expression of genes mediating the pathogenesis of DKD (Figure 43) (524). Several microRNAs and certain long noncoding RNAs also have regulatory roles in DKD, including promoting/modulating fibrotic gene expression in renal cells by targeting transcription repressors (525). Although the mechanisms of such cellular memory are not entirely elucidated, its presence is supported by the regression of morphologic lesions in diabetic kidneys after a long period of normoglycemia following pancreas transplantation (369). Similarly, the long-lasting effects of previous strict glycemic control observed in persons with type 1 diabetes in the DCCT or type 2 diabetes in the UKPDS could be attributed to cellular metabolic memory. Therapeutic approaches targeting transforming growth factor beta (TGF-β), angiotensin II type 1 receptor, or microRNAs can block some of the events involved in the pathogenesis of DKD, suggesting the need for novel therapies.
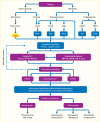
FIGURE 43.
Signaling and Epigenetic Networks Involved in the Pathogenesis of Diabetic Complications and Metabolic Memory. Metabolic and hemodynamic disorders associated with diabetes can upregulate growth factors and lipids that trigger signaling pathways, transcription (more...)
Treatment of Diabetes-Related Kidney Disease
Large, well-designed clinical trials have examined the effects of treatments on the onset and progression of kidney disease in persons with diabetes. The results of these trials helped inform and refine clinical practice guidelines for the management of persons with diabetes and kidney disease. This section summarizes the current understanding of kidney protective treatments in those with diabetes.
Glycemic Control
Hyperglycemia plays a fundamental role in the development of DKD, as discussed previously in this review. Many clinical trials have examined the effect of glycemic control on the course of DKD (250,513,527,528,529,530,531,532,533,534,535,536,537,538,539,540,541,542).
In type 1 diabetes, the DCCT and its long-term follow-up, the EDIC observational study, demonstrated that intensive treatment of hyperglycemia prevents the development of albuminuria and slows its progression (513,543). In the DCCT, 1,441 persons with type 1 diabetes were randomly divided into two groups, half receiving intensive insulin therapy and the other half receiving conventional insulin therapy. Participants were followed for a mean of 6.5 years (252). Intensive insulin therapy reduced the risks of moderate albuminuria (≥40 mg/24 hours) and severe albuminuria (≥300 mg/24 hours) by 39% and 54%, respectively. In both the primary and secondary prevention cohorts, intensive therapy reduced the proportion of participants developing moderate or severe albuminuria more than conventional treatment (Figure 44). At the end of the randomization period, both treatment groups were offered intensive therapy and followed during the EDIC study to determine the longer-term effects of prior intensive treatment on kidney disease (513). Despite the fact that the difference in A1C levels achieved during the DCCT (7.4% [57 mmol/mol] in the intensive treatment group vs. 9.1% [76 mmol/mol] in the conventional treatment group, p<0.01) disappeared promptly during the EDIC study, the beneficial effect of early intensive insulin treatment persisted for 8 years after the end of randomization, with 57% lower adjusted risk for moderate albuminuria and 84% lower risk for severe albuminuria in the former intensively treated group compared with the former conventionally treated group (Figure 45) (513). In later analyses, although the risk of treated kidney failure did not differ significantly between treatment groups during 22 years of combined follow-up (0.5 cases/1,000 person-years in the intensive treatment group vs. 1.1 cases/1,000 person-years in the conventional treatment group, p=0.10), intensive early glycemic control reduced the risk of a sustained eGFR <60 mL/min/1.73 m2 by 50% (95% CI 18%–69%, p=0.006) (532). Intensive metabolic control also reduced CVD outcomes by 42%, with a specific 57% decrease in myocardial infarction, stroke, or death from CVD; these effects were partly mediated by the reduced incidence of DKD (533).
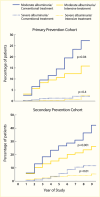
FIGURE 44.
Cumulative Incidence of Moderately Elevated and Severe Albuminuria in Participants With Type 1 Diabetes, Diabetes Control and Complications Trial. Moderate albuminuria is defined as an albumin excretion rate ≥40 mg/24 hours; severe albuminuria (more...)
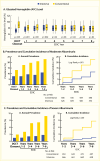
FIGURE 45.
Differences in Glycated Hemoglobin (A1C) Level and Prevalence and Incidence of Moderate and Severe Albuminuria, by Randomized Treatment Group at the End of the Diabetes Control and Complications Trial and Each Year in the Epidemiology of Diabetes Interventions (more...)
The long-term positive effect of intensive glycemic control—“metabolic memory” or the glycemic “legacy effect”—observed in persons with type 1 diabetes followed in the DCCT/EDIC study (533) has also been observed in persons with type 2 diabetes. The UKPDS was the first large-scale clinical trial to evaluate the effects of intensive glycemic control versus conventional therapy with diet alone on long-term diabetes complications in individuals with newly diagnosed type 2 diabetes and overweight or obesity. No differences in the relative risk for kidney outcomes (renal death or kidney failure) were seen between the intensive and conventional therapy groups after 10 years (544). The level of glycemic control achieved in the UKPDS was similar to that achieved in the DCCT.
Subsequent randomized controlled trials explored the effect of even more intensive glycemic control on microvascular and macrovascular outcomes in type 2 diabetes. A meta-analysis of these trials (537) indicated that even more intensive glucose lowering was associated with a significant reduction in the rate of moderate albuminuria (risk ratio 0.90, 95% CI 0.85–0.96) but had no effects on mortality, kidney failure, or other vascular outcomes. Baseline characteristics and mean A1C levels achieved during standard and intensive glycemic therapy in these clinical trials are shown in Table 17 (537,538,539,540,541,542,545). The modest gains in intermediate outcomes across these studies were counterbalanced by a twofold to threefold higher risk of severe hypoglycemia that required the intervention of another person in the intensive treatment arms of the Action to Control Cardiovascular Risk in Diabetes (ACCORD), ADVANCE, and Veterans Affairs Diabetes Trial (VADT) (Table 18) (541,542,545). The ACCORD trial, targeting an A1C <6.0% (<42 mmol/mol) in the intensive intervention arm, reported an increased risk of cardiovascular death for intensive versus conventional glycemic control, although it remains unclear whether this effect was related to more hypoglycemic episodes, the use of additional hypoglycemic medicines, or to the target glycemic level itself. Significant weight gain in those receiving intensive treatment also might have offset the beneficial effects of lower A1C levels.

TABLE 17.
Characteristics of Persons With Type 2 Diabetes Included in Randomized Controlled Studies of Intensive Versus Conventional Glycemic Treatment

TABLE 18.
Effect of Intensive Versus Conventional Glycemic Control on Kidney Outcomes in Type 2 Diabetes
Persons with advanced kidney disease experience reduced renal gluconeogenesis and impaired kidney clearance of insulin and certain oral hypoglycemic drugs (546), potentially increasing the likelihood of severe hypoglycemia, particularly when associated with inadequate nutrition. Furthermore, because A1C may underestimate the level of glycemia in uremic patients, due to the shortened lifespan of red blood cells and treatment with erythropoietin, establishing an optimal target A1C in uremic patients remains challenging (547,548). Persons with DKD often have higher glycemic levels than expected for a given A1C, albeit with a wide variation in the glucose/A1C relationship (549,550,551,552,553,554). The Dialysis Outcomes and Practice Patterns Study (DOPPS) (555) found a U-shaped association between A1C and all-cause mortality in hemodialysis patients with type 1 or type 2 diabetes. The lowest death rates were associated with A1C levels of 7.0%–7.9% (53–63 mmol/mol), comparable to those found in a population-based study of patients with diabetes and an eGFR <60 mL/min/1.73 m2 who were not on dialysis (556). Another study using time-dependent modeling of A1C values in patients with diabetes on dialysis found similar nonlinear associations with mortality (557). Nevertheless, A1C remains the best clinical marker of long-term glycemic control in persons with DKD, particularly if combined with self-monitoring or continuous monitoring of blood glucose (10). The clinical value of alternative glycemic biomarkers to A1C, including measurement of glycated albumin and fructosamine, is still being explored.
Metabolic and Inflammatory Control and Insulin-Sensitizing Therapies
Metformin is one of the most commonly prescribed therapies for management of hyperglycemia in type 2 diabetes and exerts its blood glucose-lowering effects primarily through inhibition of gluconeogenesis in the liver (558). Despite widespread use of metformin in type 2 diabetes, large-scale clinical trials with DKD-specific primary endpoints are lacking. In a small trial of 51 adults with type 2 diabetes and DKD who received a 12-week course of either the sulfonylurea glibenclamide or metformin, those who were treated with metformin exhibited a significant attenuation of albuminuria (559). The Hyperinsulinemia: The Outcome of its Metabolic Effects (HOME) study also evaluated the effects of metformin versus placebo when used in conjunction with insulin and found no significant difference in urine albumin excretion with metformin therapy (560). In A Diabetes Outcome Progression Trial (ADOPT), 4,351 recently diagnosed, drug-naïve adults with type 2 diabetes were randomized to treatment with metformin, the thiazolidinedione rosiglitazone, or the sulfonylurea glyburide for 4 years (561). Changes in the eGFR via the Modification of Diet in Renal Disease (MDRD) Study equation from baseline were +1.4% (95% CI 0.0%–2.9%) with metformin, +5.1% (95% CI 3.6%–6.7%) for rosiglitazone, and -0.4% (95% CI -2.0%–1.2%) for glyburide (p=0.0005 for metformin vs. rosiglitazone); there were no differences between treatment groups in incident moderate albuminuria, hypertension, or an eGFR <60 mL/min/1.73 m2 (561).
Glucagon-Like Peptide-1 Receptor Agonists
GLP-1 RAs are increasingly used for glycemic management in persons with type 2 diabetes. GLP-1 RAs act at the level of the seven-transmembrane G protein-coupled GLP-1 receptor to activate adenylate cyclase and stimulate a cascade resulting in the postprandial release of insulin, as well as numerous additional downstream effects, including increased beta cell proliferation, somatostatin release, natriuresis and diuresis, glucose uptake in the muscle and adipose tissue, skeletal muscle perfusion, lipolysis, and satiety, and decreased beta cell apoptosis, glucagon secretion, gastric emptying, gastrointestinal motility, gluconeogenesis, steatosis, and generalized inflammation (562).
As a drug class, GLP-1 RAs undergo renal clearance; thus, kidney effects of GLP-1 RAs are difficult to evaluate, and studies are largely limited to intermediate health outcomes, including changes in the eGFR or albuminuria (563,564,565), presence of new-onset severe albuminuria (566,567), time to new-onset severe albuminuria (568), or persistent severe albuminuria (9).
Most existing data regarding kidney outcomes in GLP-1 RA studies come from large, placebo-controlled safety and cardiovascular outcomes trials in adults with type 2 diabetes who either have preexisting CVD or are at high risk for CVD (Table 19) (8,9,567,569,570,571,572,573). Both the Trial to Evaluate Cardiovascular and Other Long-term Outcomes with Semaglutide in Subjects with type 2 diabetes (SUSTAIN-6) and the Liraglutide Effect and Action in Diabetes: Evaluation of Cardiovascular Outcome Results (LEADER) trial enrolled individuals with type 2 diabetes and prevalent CVD and showed significantly lower risk for new or worsening DKD with semaglutide (HR 0.64, 95% CI 0.46–0.88, p=0.005) or liraglutide (-22%, HR 0.74, 95% CI 0.60–0.91) versus placebo (8,9). However, both the SUSTAIN-6 and LEADER trials used a prespecified composite kidney outcome, including persistent doubling of serum creatinine concentration, new onset of persistent macroalbuminuria, an eGFR ≤45 mL/min/1.73 m2, need for continuous kidney replacement therapy, and death from kidney disease (8,9), rather than direct assessments of kidney function. The Exenatide Study of Cardiovascular Event Lowering (EXSCEL) also used a composite score, including 40% eGFR decline, use of kidney replacement therapy, kidney death, or new macroalbuminuria, to evaluate the effects of a median of 3.2 years of treatment with extended-release exenatide versus placebo in people with type 2 diabetes. EXSCEL found nonsignificant improvement in the composite kidney outcome with GLP-1 RA therapy (HR 0.88, 95% CI 0.76–1.01, p=0.065) and a similar effect on worsening kidney function (HR 0.88, 95% CI 0.74–1.05, p=0.16) (570,571).

TABLE 19.
Characteristics of Participants in Studies Assessing Kidney Outcomes and Use of Glucagon-Like Peptide-1 Receptor Agonists, Sodium-Glucose Cotransporter-2 Inhibitors, and Dipeptidyl Peptidase-4 Inhibitors
Dedicated kidney outcomes trials, particularly in individuals with known kidney disease, are limited but have confirmed the positive effects of GLP-1 RAs seen in safety and efficacy trials. In the Study Comparing Dulaglutide with Insulin Glargine on Glycemic Control in Participants with Type 2 Diabetes and Moderate or Severe Chronic Kidney Disease (AWARD-7), dulaglutide reduced severe albuminuria by 29% (95% CI 11.5%–43.0%) and attenuated eGFR decline versus insulin glargine alone in adults with type 2 diabetes and moderate to severe CKD (564). In the Evaluate Renal Function with Semaglutide Once Weekly (FLOW) trial, patients with type 2 diabetes and CKD (defined by an eGFR 50–75 mL/min/1.73 m2 and urinary ACR 300–5,000 mg/g or an eGFR 25–<50 mL/min/1.73 m2 and urinary ACR 100–5,000 mg/g) randomized to semaglutide had a 21% lower risk for the composite kidney-specific outcomes (HR 0.79, 95% CI 0.66–0.94) and a 29% lower risk for cardiovascular death (HR 0.71, 95% CI 0.56–0.89) compared with placebo (573).
These trials confirm the favorable effects of GLP-1 RAs on kidney, cardiovascular, and survival outcomes in high-risk populations with type 2 diabetes, although gastrointestinal disorders are common causes of treatment discontinuation.
Sodium-Glucose Cotransporter-2 Inhibitors
SGLT2 inhibitors have revolutionized the treatment of cardiovascular and kidney outcomes in type 2 diabetes (6,7). Several large randomized controlled trials have documented the efficacy of SGLT2 inhibitors in improving kidney outcomes in adults with type 2 diabetes (Table 19) (574,575,576,577,578,579,580,581,582,583,584,585,586,587,588). The Empagliflozin, Cardiovascular Outcome Event Trial in Type 2 Diabetes Mellitus Patients (EMPA-REG) enrolled 7,020 participants age >18 years with type 2 diabetes and an eGFR >30 mL/min/1.73 m2 to assess the cardiovascular safety of empagliflozin (574). A secondary analysis of trial data noted that individuals randomized to treatment with empagliflozin had a 39% reduction in their risk for worsening DKD (incident severe albuminuria, doubling of serum creatinine, or need for dialysis/transplant) (575). The Canagliflozin Cardiovascular Assessment Study (CANVAS) and Canagliflozin and Renal Events in Diabetes with Established Nephropathy Clinical Evaluation (CREDENCE) trials enrolled adults with type 2 diabetes and an eGFR >30 mL/min/1.73 m2 (CREDENCE excluded persons with an eGFR >90 mL/min/1.73 m2) and documented a similar efficacy of canagliflozin (576,578). Both trials demonstrated a 30%–40% reduction in risk for most adverse kidney outcomes, including worsening albuminuria, >40% decline in the eGFR, and dialysis dependence.
Although the kidney effects of SGLT2 inhibitors observed in type 2 diabetes trials may also apply to people with type 1 diabetes, clinical trials that included patients with type 1 diabetes have not shown the same unequivocal effects on preventing kidney outcomes (589). Further, the increased risk of euglycemic diabetic ketoacidosis is a limiting factor for using SGLT2 inhibitors as adjunctive therapy in people with type 1 diabetes. Strategies to mitigate the risk for euglycemic ketoacidosis, such as continuous ketone monitors, may facilitate the safe prescribing of SGLT2 inhibitors for people with type 1 diabetes.
Mineralocorticoid Receptor Antagonists
Excessive mineralocorticoid receptor (MR) activation with resultant inflammation and fibrosis has been implicated in the pathogenesis of DKD (590). Likewise, murine MR knockout models document protection against kidney inflammation and fibrosis (591). In the Chronic Renal Insufficiency Cohort (CRIC), higher serum aldosterone concentrations were associated with kidney disease progression in adults with and without type 2 diabetes (592). In the Finerenone in Reducing Kidney Failure and Disease Progression in DKD (FIDELIO-DKD) clinical trial, finerenone, a nonsteroidal MRA, attenuated risk of DKD progression (sustained decrease in the eGFR ≥40% from baseline) by 19% (HR 0.81, 95% CI 0.72–0.92) in adults with type 2 diabetes (593). The Finerenone in Reducing Cardiovascular Mortality and Morbidity in DKD (FIGARO-DKD) clinical trial found similar effects on DKD progression (HR 0.87, 95% CI 0.75–1.00) (594). Data on MRA treatment for persons with type 1 diabetes are limited (595).
Endothelin Receptor Antagonists
Inappropriate endothelin receptor activation is thought to contribute to CKD. Endothelin receptor antagonists (ERA) primarily target the ETA receptor and have demonstrated kidney-protective effects. The ERA atrasentan attenuated progression of DKD in adults with type 2 diabetes in the Study of Diabetic Nephropathy with Atrasentan (SONAR) trial (596). Unlike SGLT2 inhibitors, ERAs increase sodium and fluid retention, resulting in edema and increased risk of developing heart failure (597,598). This effect has limited the development and widespread use of agents in this class, especially in people who are predisposed to volume overload, including individuals with DKD. Indeed, a large randomized controlled trial in patients with type 2 diabetes and DKD using a relatively nonselective ERA avosentan was terminated early due to increased rates of heart failure (597). Additionally, a higher rate (3.5% vs. 2.6%) of heart failure hospitalization with atrasentan was observed in the SONAR trial despite the use of diuretics and exclusion of patients with heart failure or high B-type natriuretic peptide (BNP) concentrations (596). Accordingly, while ERAs hold promise as a therapy for DKD, it is unclear whether benefits would outweigh risks, and ERAs are likely best used in combination with a natriuretic or diuretic agent, such as an SGLT2 inhibitor. No ERA trial data in persons with type 1 diabetes are available as of July 2024.
Blood Pressure Control
Blood pressure control, particularly with RAAS inhibitors, significantly decreases the risk of progression from moderate albuminuria to severe albuminuria, increases the rate of regression from moderate albuminuria to normoalbuminuria, and decreases the risk of heart failure and overall cardiovascular outcomes in persons with DKD, regardless of type of diabetes (599,600,601). Although several types of antihypertensive drugs are effective in ameliorating the course of DKD, including beta blockers, non-dihydropyridine calcium channel blockers, diuretics, and RAAS inhibitors, the purported relationship between increased intraglomerular pressure and proteinuria that is mediated by RAAS components, as well as their association with virtually every aspect of kidney disease progression, has been borne out by evidence that RAAS inhibitors, including primarily ACE inhibitors and ARBs, prevent progression of DKD.
A landmark study of 409 mostly hypertensive persons with type 1 diabetes and urinary protein excretion ≥500 mg/24 hours, who were randomized to receive either captopril (an ACE inhibitor) or placebo, found a 48% lower risk of doubling of serum creatinine concentration in the captopril group compared to the placebo group after a median follow-up of 3 years (Figure 46) (602). The risk of the combined endpoints of death, dialysis, or transplantation was 50% lower (Figure 46). A significant renoprotective effect of captopril, however, was limited to those with baseline serum creatinine concentrations ≥1.5 mg/dL. No long-term studies have examined the renoprotective efficacy of ARBs in type 1 diabetes and advanced kidney disease. In persons with type 1 diabetes and lower levels of ACR, with or without hypertension, randomized controlled studies demonstrate that RAAS inhibitors do not prevent increases in ACR, serum creatinine, or kidney failure during up to 5 years of follow-up (Table 20) (542,602,603,604,605,606,607,608,609,610,611,612,613,614,615,616,617,618,619). Moreover, when given to normoalbuminuric, normotensive persons with type 1 diabetes, these treatments do not reduce the rate of expansion of the fractional mesangial volume over a period of 4–5 years (603).
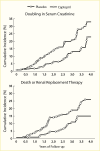
FIGURE 46.
Effect of Captopril on Incidence of Kidney Disease in Persons With Type 1 Diabetes and Proteinuria, 1987–1992. The Collaborative Study included 409 subjects with type 1 diabetes and urinary protein excretion ≥500 mg/24 hours, who were (more...)

TABLE 20.
Randomized Controlled Studies of Renin-Angiotensin System Inhibitors
In hypertensive persons with type 2 diabetes and severe albuminuria, the RENAAL study (612) and the Irbesartan Diabetic Nephropathy Trial (IDNT) (613) reported results that were similar to those in persons with type 1 diabetes and severe albuminuria (Table 20) (602). Both studies reported lower risks of doubling of serum creatinine and kidney failure compared with placebo; the IDNT found a 39% lower risk of doubling of serum creatinine compared with amlodipine. The Irbesartan in Patients With Type 2 Diabetes and Microalbuminuria (IRMA 2) study found that high-dose irbesartan prevented progression to severe albuminuria in hypertensive persons (611). Although none of these three ARB trials found significant reductions in kidney failure incidence during the intervention periods, several cost-benefit analyses based on the IDNT and the cost of medicine and kidney failure treatment specific to the United States, Canada, and several European countries consistently predicted both long-term survival advantage and higher cost-effectiveness with ARB than with other treatments (620). These benefits might be due in part to less comorbidity associated with RAAS treatment, such as heart failure and retinopathy, and in part to lower kidney failure incidence when longer time periods are considered. No long-term studies have examined the renoprotective efficacy of ACE inhibitors in persons with type 2 diabetes and ACR ≥300 mg/g.
Dual RAAS blockade is as effective as monotherapy in reducing blood pressure and albuminuria in persons with diabetes (616,621,622), but it does not offer benefit and has more adverse effects, so it is not recommended (614). The Ongoing Telmisartan Alone and in Combination With Ramipril Global Endpoint Trial (ONTARGET) (619) and Veterans Affairs Nephropathy in Diabetes (VA NEPHRON-D) trial (623) found an excess risk of acute kidney injury and hyperkalemia with dual RAAS therapy, without a significant cardiovascular and renal benefit. Further, even after 5 years of treatment, albuminuria increased to pretreatment levels soon after the withdrawal of these drugs (603,604,605). This observation suggests the need for longer periods of normalization of both ACR and glycemic levels to achieve a durable treatment effect on underlying disease processes or more sensitive early markers of kidney disease progression. Adding the direct renin inhibitor aliskiren to an ARB (losartan) in persons with type 2 diabetes, hypertension, and ACR ≥300 mg/g more effectively reduced mean urinary ACR than losartan alone (20%, 95% CI 9%–30%, p<0.001) (624); the mean eGFR decline during the 24-week study period also tended to be lower in the aliskiren group (2.4 mL/min/1.73 m2 vs. 3.8 mL/min/1.73 m2 in the placebo group, p=0.07). The Aliskiren Trial in Type 2 Diabetes Using Cardiorenal Endpoints (ALTITUDE), a subsequent, larger study in persons with type 2 diabetes, CKD, and high cardiovascular risk, was stopped early due to therapeutic futility and increased risk of stroke and other adverse events (625). Neither ALTITUDE nor VA NEPHRON-D was continued long enough to establish efficacy, and the trials remained underpowered for their primary CVD and renal outcomes. Based on their other findings, however, dual RAAS blockade is contraindicated due to safety concerns (10).
In a meta-analysis exploring the benefit of intensive (achieved systolic blood pressure ≤135 mmHg) versus standard (achieved systolic blood pressure ≤140 mmHg) blood pressure targets in subjects with type 2 diabetes, the former was associated with significant 17% and 27% reductions in the odds for moderate and severe albuminuria, respectively (626). For persons with severe albuminuria (>3 g/24 hours), a post hoc analysis from the MDRD study indicated better kidney outcomes with a lower blood pressure goal (<130/80 mmHg) (627). Whereas stringent blood pressure targets lowered the risk for stroke and death, the few studies reporting kidney failure or doubling of serum creatinine showed no benefits for these outcomes, suggesting the importance of individualizing blood pressure goals in persons with diabetes to account for the presence and severity of CKD, age, and associated cardiovascular risk. Moreover, ACE inhibitors and beta blockers may be less effective in controlling blood pressure and reducing albuminuria in Black than in White persons (628,629,630), suggesting that different drug combinations may be more effective in slowing progression of kidney disease in Black persons (631,632).
Table 21 shows the Eighth Joint National Committee’s (JNC 8) recommendations for management of hypertension in the population with diabetes and CKD in comparison with other guidelines (17,632,633,634,635). The thresholds and goals recommended by the guideline-writing groups differ within a narrow range, with 2014–2015 JNC 8 recommendations endorsing less intensive and more individualized blood pressure targets for diabetes and CKD than more recent guidelines. In elderly persons, in particular, antihypertensive treatment should be individualized, taking into consideration such factors as frailty, comorbidities, and albuminuria (Figure 47) (632). There is consensus on the initial use of RAAS treatment in persons with albuminuria, but not for those without elevated albuminuria.

TABLE 21.
Guideline Comparisons of Blood Pressure Goals and Drug Therapy for Adults With Hypertension

FIGURE 47.
2014 Hypertension Guideline Management Algorithm, Eighth Joint National Committee. ACEI, angiotensin-converting enzyme inhibitor; ARB, angiotensin receptor blocker; CCB, calcium channel blocker; CKD, chronic kidney disease; DBP, diastolic blood pressure; (more...)
Control of Blood Lipids
Persons with diabetes and CKD typically have an atherogenic lipid profile, characterized by low concentrations of HDL cholesterol and high concentrations of small, dense LDL cholesterol particles and triglycerides (402,636,637,638). Treatment with lipid-lowering medicines, particularly statins, can reduce the high cardiovascular risk in this population. There is no conclusive evidence, however, that such treatment also affects progression of DKD (639,640,641,642).
The Study of Heart and Renal Protection (SHARP) (643) is the largest randomized controlled trial in persons with CKD (average eGFR 27 mL/min/1.73 m2) to demonstrate that a lipid-lowering strategy with a statin plus ezetimibe significantly reduces major atherosclerotic events, such as coronary death, myocardial infarction, nonhemorrhagic stroke, and arterial revascularization procedures, compared with placebo (HR 0.83, 95% CI 0.74–0.94). The relative effect on these outcomes was similar in persons with or without diabetes, although the study was not adequately powered for subgroup analyses—only 23% of the 9,438 participants had diabetes. The study detected no effect of lipid-lowering treatment on the frequency of doubling of the baseline serum creatinine concentration or progression to kidney failure. SHARP and other studies (644,645,646) found no cardiovascular benefit of statin therapy when it was initiated in persons with diabetes after the onset of dialysis. By contrast, lipid-lowering therapy appears highly beneficial in reducing cardiovascular events in persons with and without diabetes with a functioning kidney transplant (647).
Other lipid-lowering medicines, such as gemfibrozil and fenofibrate, may be of value in the management of cardiovascular outcomes or new-onset albuminuria in persons with DKD (648).
Dietary Modification
In animals with experimental diabetes, reduced protein intake protects against hyperfiltration and progressive sclerosis of functioning glomeruli (649,650). In persons with type 1 diabetes and normal urinary albumin excretion, short-term dietary protein restriction favorably modifies glomerular hemodynamic function, and in those with moderate albuminuria, it also reduces urinary albumin excretion (651,652,653,654,655). Similar effects have been found in persons with clinical proteinuria (Table 22) (656,657,658,659). The largest study (660) randomized 82 persons with type 1 diabetes, severe albuminuria, and prerandomization eGFR decline of 7.1 mL/min/year to usual-protein diet (1.02 g/kg/day, 95% CI 0.95–1.10) or reduced-protein diet (0.89 g/kg/day, 95% CI 0.83–0.95) (p=0.005) for a period of 4 years. At the end of the study, the CVD-adjusted risk of kidney failure or death was 0.23 (95% CI 0.07–0.72, p=0.01) in the group receiving the reduced-protein diet compared with the group receiving the usual diet (Figure 48), suggesting that even moderate protein restriction, which moves intakes from excess toward the norm, provides benefits beyond conventional drug therapy in these persons.

TABLE 22.
Clinical Trials of the Effect of Dietary Protein Reduction on the Course of Diabetic Nephropathy in Persons With Type 1 Diabetes and Severe Albuminuria
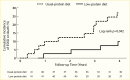
FIGURE 48.
Cumulative Incidence of End-Stage Kidney Disease or Death in Persons With Type 1 Diabetes, by Protein Intake. Eighty-two persons with type 1 diabetes and severe albuminuria were randomized to usual-protein diet and low-protein diet. The numbers at the (more...)
Fewer studies are available in persons with type 2 diabetes. A study of the efficacy of dietary protein restriction in 160 persons with type 2 diabetes and moderate albuminuria or ≥5 years duration of diabetes found no beneficial effect of protein restriction on albuminuria or the eGFR during a mean follow-up of 28 months (661). In fact, a protein intake restricted to 0.8 g/kg/day could not be effectively achieved beyond the first 6 months of the study, reflecting the inconvenience associated with a strict dietary regimen that may outweigh the benefits of such therapy.
A systematic review of randomized studies (662) concluded that a reduced-protein diet in persons with CKD associated with either type of diabetes had no significant effect on kidney function relative to normal protein consumption, despite improvements in proteinuria and A1C, and that dietary protein restriction may significantly increase the risk for malnutrition in those with more advanced CKD (663). On the other hand, studies evaluating a reduction or alteration of protein intake in persons with DKD are generally small (under 100 subjects), of short duration (<2 years), have limited documentation on the quality of protein (animal or vegetable), fat and carbohydrate intake, and evaluate intermediate outcomes, i.e., albuminuria and the eGFR, rather than major health outcomes. Because the low quality of evidence precludes a recommendation, KDIGO suggests maintaining 0.8 g/kg/day dietary protein intake for persons with DKD not treated with dialysis (10).
Sodium intake is a major contributor to blood pressure increase. According to the Institute of Medicine (664), about 75% of dietary sodium intake is obtained from preprocessed foods or is added to restaurant food during preparation, and only about 25% comes from natural sources or is added by the consumer. The Dietary Guidelines for Americans (665) recommend that persons age ≥51 years, Black persons, and persons with hypertension, diabetes, or CKD limit their sodium intake to 1,500 mg daily. In the NHANES 2005–2008, virtually all (99.4%) of those encouraged to limit their sodium intake exceeded the recommended limit on a daily basis (666).
Although sodium reduction has a positive impact on blood pressure in persons with diabetes (667), its long-term effect on kidney outcomes remains unclear. Most individuals may benefit from a Dietary Approaches to Stop Hypertension (DASH) diet, a reduced-sodium diet emphasizing fruits, vegetables, low-fat dairy foods, whole grains, nuts, poultry, fish, and smaller amounts of red meat and refined sugars than the typical diet in the United States (668). The DASH diet reflects a shift towards whole-diet approaches to the management of DKD that integrate multiple healthful eating goals simultaneously, without a focus on single nutrients. In addition to lowering blood pressure, a DASH-type diet was associated with preserved kidney function over 11 years in women from the Nurses’ Health Study with a mildly decreased eGFR, an effect primarily associated with reduced red meat intake (669). Nonetheless, the DASH diet may not be suitable, without modification, for persons with an eGFR <60 mL/min/1.73 m2 because of its high content of potassium (4.5 g/day), phosphorus (1.7 g/day), and protein (1.4 g/kg/day).
Other Treatments
Modification of blood pressure, metabolic control, diet, and treatment with dialysis or transplantation are the mainstays of treatment of kidney disease in persons with diabetes, and a majority of the research into new therapeutic approaches has focused on one or more of these therapies. Nevertheless, a growing body of evidence indicates that the development of DKD is related to specific metabolic derangements induced by hyperglycemia that interplay with hemodynamic pathways. Advanced glycation endproducts (670), TGF-β, and other growth factors (671,672,673,674) play key roles in promoting chemical, cellular, and tissue disorders linked to progression of kidney disease. Animal studies suggest that inhibitors of advanced glycation endproduct formation ameliorate glomerulosclerosis and improve albuminuria and kidney function (675), and advanced glycation endproduct crosslink breakers improve endothelial function and arterial compliance, with reductions in arterial pulse pressure and albuminuria in persons with type 1 diabetes (676,677). Antioxidant inflammation modulators, such as eicosapentaenoic acid or pentoxifylline (678,679), improved kidney function and proteinuria in small studies of persons with type 1 or type 2 diabetes, and treatment with Rho GTPase and Rho-associated kinase inhibitors (ROCKs) mitigated mesangial expansion, thickening of glomerular basement membrane, and albuminuria in experimental DKD (680).
Persons with DKD require multidisciplinary management involving a combination of all treatments and behavioral adjustments to hold off the progression of kidney disease per se and to prevent the associated complications. The Steno-2 study, a landmark prospective, randomized trial in Denmark (681), demonstrated that intensive multifactorial intervention reduces the incidence of microvascular and macrovascular outcomes in patients with type 2 diabetes and persistent moderate albuminuria. At the end of the randomization period (mean duration 7.8 years), participants in the intervention arm (n=80) had significantly lower systolic and diastolic blood pressure, A1C, total cholesterol, LDL cholesterol, triglycerides, and ACR than those randomized to conventional treatment (n=80). Although these differences disappeared during the 5.5 years of post-trial observational follow-up, intensive therapy reduced the risk of death (HR 0.54, 95% CI 0.32–0.89, p=0.02), death from CVD (HR 0.43, 95% CI, 0.19–0.94, p=0.04), severe albuminuria (HR 0.44, 95% CI 0.25–0.77, p=0.04), diabetic retinopathy (HR 0.57, 95% CI 0.37–0.88, p=0.01), and autonomic neuropathy (HR 0.53, 95% CI 0.34–0.81, p=0.004) during the entire 13.3-year study period.
Treatment of Diabetes-Related Kidney Disease in Youth
Despite the increasing frequency of both type 1 and type 2 diabetes in youth and the particularly aggressive course of DKD in youth with type 2 diabetes, few clinical trials have evaluated DKD outcomes in youth. TODAY is one such clinical trial that focused specifically on treatment of youth with type 2 diabetes. In TODAY, 699 youth age 10–18 years with type 2 diabetes for <2 years were randomized to metformin monotherapy, metformin plus rosiglitazone, or metformin plus an intensive lifestyle intervention (16,44). Incidence rates of moderate albuminuria increased across all treatment groups during an average follow-up of 3.9 years (overall incidence rate increase from 6.3% at baseline to 16.6% at trial completion) (682). In the long-term follow-up after the end of randomization, 60.1% of participants had at least one microvascular complication and 28.4% had at least two diabetes complications at a mean age of 26.4 years (44). Compared with non-Hispanic White youth with type 2 diabetes, Hispanic youth had a 50% higher risk of microvascular complications (HR 1.50, 95% CI 1.13–2.00), similar to Black youth (HR 1.46, 95% CI 1.09–1.96) (44). Adherence to treatments was a challenge in this trial, with only 54% of participants in the lifestyle intervention group meeting targets. Indeed, reduced adherence to medication, lifestyle interventions, and self-monitoring in youth compared with adults may be responsible, in part, for the more aggressive course of DKD in youth (683,684). Factors such as depression, living in a single-parent home, and poverty may be key determinants of low adherence. In the TODAY cohort, 70% of the youth lived in a single-parent household, and 42% lived in households with an annual income less than $25,000 (685).
There is considerable interest in assessing the benefits of SGLT2 inhibitors in young persons, given their remarkable efficacy in adults. Nevertheless, evidence for the tolerability and efficacy of SGLT2 inhibitors in youth with type 2 diabetes is limited. Pharmacokinetic and single-dose studies in youth with type 2 diabetes have documented a dose-dependent increase in urinary glucose excretion (686) and an eGFR dip similar to what has been observed in short-term treatment in adults with type 2 diabetes (687).
Currently, no SGLT2 inhibitors are approved by the U.S. Food and Drug Administration (FDA) for use in persons age <18 years. Likewise, thiazolidinediones have not been approved for youth-onset type 2 diabetes, despite their beneficial effects on insulin sensitivity that could benefit insulin-resistant adolescents. The FDA has approved two GLP-1 RAs for the treatment of type 2 diabetes in youth age 10–17 years, including liraglutide 1.8 mg/day and exenatide extended-release injections. With only limited data available on treatment with MRAs in youth and no studies of ERAs, much remains to be done to establish efficacious therapeutic options in children and adolescents with diabetes and DKD.
Other Kidney Diseases Associated With Infections in Persons With Diabetes
Urinary Tract Infections
Persons with diabetes may be more susceptible to infections of the urinary tract. Autopsy studies from the pre-antibiotic era (688,689,690,691,692) reported 10%–20% prevalence of histologic pyelonephritis in persons with diabetes, five times that in persons without diabetes. Not only was the frequency of urinary tract infection greater in those with diabetes at that time, but the infections were often more serious and protracted (689). With the introduction of effective antimicrobial therapy, the frequency and severity of urinary tract infections may have diminished (693).
Table 23 presents the prevalence of asymptomatic bacteriuria in persons with and without diabetes from several different clinic- or hospital-based populations (694,695,696,697,698,699,700,701,702,703,704,705,706,707,708,709,710,711). In a systematic review and meta-analysis of published data since 1966 (694), women with diabetes have about three times the frequency of bacteriuria as women without diabetes (OR 2.6, 95% CI 1.6–4.1), and men with diabetes have about four times the frequency of bacteriuria as healthy control subjects (OR 3.7, 95% CI 1.3–10.2) (694,699,700,704,712,713,714,715,716,717,718,719). Some studies have reported a longer duration of diabetes in women with asymptomatic bacteriuria than in those without (pooled difference 0.17 years, 95% CI 0.03–0.31, p=0.01), but the same review found no difference in A1C (694). Some studies have shown a relationship between asymptomatic bacteriuria in persons with diabetes and more frequent development of genitourinary tract infections (711,720,721,722,723), but others have not found a relationship (693,724). In most studies, the microorganisms causing asymptomatic bacteriuria in persons with diabetes are similar to those causing bacteriuria in persons without diabetes (693), but a survey of 514 persons with diabetes and 405 persons without diabetes found that nearly one-half of those with diabetes and bacteriuria were infected by bacteria other than E. coli, whereas all but one case of bacteriuria in persons without diabetes were caused by E. coli (713). The prevalence of asymptomatic bacteriuria is not influenced by the type of diabetes (693,694).

TABLE 23.
Prevalence of Asymptomatic Bacteriuria in Populations With and Without Diabetes
The reported prevalences of cystitis and pyelonephritis in 550 women with type 1 diabetes in the UroEDIC study of EDIC year 10 subjects surveyed for urologic complications of type 1 diabetes were 15% and 3%, respectively, during the preceding 12 months (725). Table 24 presents risk factors for urinary tract infections in this cohort. The prevalence of cystitis in women in the UroEDIC was similar to that in White women without diabetes age 20–59 years from the NHANES III. Among men in the UroEDIC, the prevalence of urinary tract infections was 4% for cystitis and 0% for pyelonephritis, too low to be included in the risk factor evaluation.

TABLE 24.
Adjusted Odds Ratios for Cystitis or Pyelonephritis in Women With Type 1 Diabetes Participating in the Uro-EDIC Survey, 2002–2004
HIV
Diabetes and hypertension associated with human immunodeficiency virus (HIV) infection are described as risk factors for the development of HIV-associated immune complex kidney disease (726,727,728). While hypertension may be a consequence of HIV-related kidney disease, the association with diabetes appears to be mediated by postinfectious glomerulonephritis (726,729). A cross-sectional comparison of persons with type 2 diabetes with and without HIV and persons with HIV only is presented in Table 25 (730). Among the 73 persons with HIV and type 2 diabetes, the combination of diabetes, higher HIV viral load, and antiretroviral treatment with abacavir was a significant predictor of ACR ≥30 mg/g. Antiretroviral therapy has nephrotoxic effects, including tubulointerstitial nephropathies, proximal tubular dysfunction, Fanconi’s syndrome, and nephrogenic diabetes insipidus (731).

TABLE 25.
Demographic and Clinical Characteristics of Subjects With HIV, Diabetes, and Both HIV and Diabetes, 2007–2009
Severe Acute Respiratory Syndrome Coronavirus 2: SARS‐CoV‐2 (COVID-19)
Kidney injury, presenting as nephritis, acute or chronic kidney injury, and transplant rejection, is commonly associated with severe acute respiratory syndrome coronavirus-2 (SARS-CoV-2) infection (coronavirus disease of 2019 [COVID-19]) and with severe outcomes (732). Patients hospitalized with COVID-19 were twice as likely to develop acute kidney injury (incidence reported between 0.5% and 42%) (733), with high mortality, particularly when requiring dialysis.
Among Medicare beneficiaries, significantly more patients with CKD were diagnosed with COVID-19 than those without CKD. Black and Hispanic patients were particularly affected during the 2020–2021 pandemic (732), with the highest rates of infection, hospitalization, and death among racial and ethnic groups.
Several mechanisms have been proposed to explain the association between COVID-19 and kidney disease, including RAAS activation, hyperglycemia-induced endothelial dysfunction, immune-mediated kidney disease, collapsing focal and segmental glomerulosclerosis, vasculitis, and IgA nephropathy, although these are not specific to DKD (733,734,735). APOL1 genotyping studies suggest that patients with COVID-19 and high-risk APOL1 alleles have significantly higher odds of collapsing glomerulopathy and acute kidney injury requiring dialysis (736,737).
Conclusions
CKD is a public health problem that affects nearly 14% of the U.S. population and is disproportionately distributed among minority and disadvantaged groups. Most risk factors for CKD are modifiable; therefore, public health strategies targeting these factors may significantly reduce the disease burden.
Diabetes is the leading cause of CKD, and the increasing prevalence of diabetes together with improved availability of dialysis and transplants have sustained a continued rise in the proportion of CKD attributable to diabetes since the 1980s. In persons with type 1 diabetes, the incidence of CKD has declined in parallel with a significant trend for earlier initiation of antihypertensive treatment following the onset of diabetes, expansion of RAAS inhibitor usage, and sustained improvements in glycemic control. On the other hand, in persons with type 2 diabetes, the incidence of CKD does not appear to be declining, possibly due to the higher number and prevalence of CKD risk factors associated with type 2 diabetes that outweigh current treatment options or their effectiveness. An ever-increasing number of persons with diabetes, 91% of whom have type 2 diabetes, require renal replacement therapy, at enormous cost to patients, their families, and society.
Improved management of hyperglycemia, hypertension, hyperlipidemia, and albuminuria have dramatically slowed progression to kidney failure, as illustrated by a decreased incidence of kidney failure attributed to diabetes since 2005. Trends in the incidence of kidney failure due to diabetes, however, differ broadly by age and race and ethnicity. At the national level, the incidence of kidney failure in persons with a primary diagnosis of diabetes remains higher in Black, Mexican American, Asian, and American Indian persons than in White persons, with the highest rates being found in Black and American Indian persons.
List of Abbreviations
- A1C
glycated hemoglobin
- ACCORD
Action to Control Cardiovascular Risk in Diabetes
- ACE
angiotensin-converting enzyme
- ACR
albumin-to-creatinine ratio
- ADA
American Diabetes Association
- ADVANCE
Action in Diabetes and Vascular Disease: Preterax and Diamicron Modified Release Controlled Evaluation
- AER
albumin excretion rate
- ARB
angiotensin receptor blocker
- BMI
body mass index
- CI
confidence interval
- CKD
chronic kidney disease
- COVID-19
coronavirus disease of 2019
- CVD
cardiovascular disease
- DASH
Dietary Approaches to Stop Hypertension
- DCCT
Diabetes Control and Complications Trial
- DKD
diabetes-related kidney disease
- DNA
deoxyribonucleic acid
- EDC
Epidemiology of Diabetes Complications study
- EDIC
Epidemiology of Diabetes Interventions and Complications study
- EDTA
ethylenediaminetetraacetic acid
- eGFR
estimated glomerular filtration rate
- ERA
endothelin receptor antagonist
- GFR
glomerular filtration rate
- GLP-1
glucagon-like peptide-1
- GLP-1 RA
glucagon-like peptide-1 receptor agonist
- HDL
high-density lipoprotein
- HIV
human immunodeficiency virus
- HR
hazard ratio
- IDNT
Irbesartan Diabetic Nephropathy Trial
- KDIGO
Kidney Disease: Improving Global Outcomes
- KEEP
Kidney Early Evaluation Program
- LDL
low-density lipoprotein
- MDRD
Modification of Diet in Renal Disease study
- MR
mineralocorticoid receptor
- MRA
mineralocorticoid receptor antagonist
- NHANES
National Health and Nutrition Examination Survey
- NKF
National Kidney Foundation
- NSAID
nonsteroidal anti-inflammatory drug
- OR
odds ratio
- RAAS
renin-angiotensin-aldosterone system
- RENAAL
Reduction of Endpoints in Non-insulin dependent diabetes with the Angiotensin II Antagonist Losartan
- SGLT2
sodium-glucose cotransporter-2
- SMR
standardized mortality ratio
- TGF-β
transforming growth factor beta
- TODAY
Treatment Options for Type 2 Diabetes in Adolescents and Youth trial
- UKPDS
United Kingdom Prospective Diabetes Study
- UroEDIC
ancillary study of urologic complications in the DCCT/EDIC cohort
- USRDS
United States Renal Data System
- VLDL
very low-density lipoprotein
Conversions
A1C: (% x 10.93) - 23.50 = mmol/mol
Albumin-to-creatinine ratio: mg/g x 0.113 = mg/mmol
Cholesterol: mg/dL x 0.0259 = mmol/L
Glucose: mg/dL x 0.0555 = mmol/L
Triglycerides: mg/dL x 0.0113 = mmol/L
Acknowledgment
This is an update of: Pavkov ME, Collins AJ, Coresh J, Nelson RG: Kidney Disease in Diabetes. Chapter 22 in Diabetes in America, 3rd ed. Cowie CC, Casagrande SS, Menke A, Cissell MA, Eberhardt MS, Meigs JB, Gregg EW, Knowler WC, Barrett-Connor E, Becker DJ, Brancati FL, Boyko EJ, Herman WH, Howard BV, Narayan KMV, Rewers M, Fradkin JE, Eds. Bethesda, MD, National Institutes of Health, NIH Pub No. 17-1468, 2018, p. 22.1–22.84.
Article History
Received in final form on June 6, 2024.
Disclaimer
The findings and conclusions in this report are those of the authors and do not necessarily represent the official position of the National Institutes of Health nor the Centers for Disease Control and Prevention.
References
- 1.
- Pavkov ME, Collins AJ, Coresh J, Nelson RG. Kidney disease in diabetes. In: Cowie CC, Casagrande SS, Menke A, et al, eds. Diabetes in America. eBook. 3rd ed. National Institute of Diabetes and Digestive and Kidney Diseases; 2018:chap 22. https://www
.ncbi.nlm .nih.gov/books/NBK568002/ - 2.
- Delgado C, Baweja M, Crews DC, et al. A unifying approach for GFR estimation: recommendations of the NKF-ASN Task Force on Reassessing the Inclusion of Race in Diagnosing Kidney Disease. Am J Kidney Dis. 2022;79(2):268-288 e1. doi:10.1053/j.ajkd.2021.08.003 [PubMed: 34563581] [CrossRef]
- 3.
- Inker LA, Eneanya ND, Coresh J, et al. New creatinine- and cystatin C-based equations to estimate GFR without race. N Engl J Med. 2021;385(19):1737-1749. doi:10.1056/NEJMoa2102953 [PMC free article: PMC8822996] [PubMed: 34554658] [CrossRef]
- 4.
- Saulnier PJ, Looker HC, Mauer M, et al. Intraglomerular dysfunction predicts kidney failure in type 2 diabetes. Diabetes. 2021;70(10):2344-2352. doi:10.2337/db21-0154 [PMC free article: PMC8576505] [PubMed: 34257069] [CrossRef]
- 5.
- Stefansson VTN, Nair V, Melsom T, et al. Molecular programs associated with glomerular hyperfiltration in early diabetic kidney disease. Kidney Int. 2022;102(6):1345-1358. doi:10.1016/j.kint.2022.07.033 [PMC free article: PMC10161735] [PubMed: 36055599] [CrossRef]
- 6.
- Braunwald E. SGLT2 inhibitors: the statins of the 21st century. Eur Heart J. 2022;43(11):1029-1030. doi:10.1093/eurheartj/ehab765 [PubMed: 34741610] [CrossRef]
- 7.
- de Boer IH, Kahn SE. SGLT2 inhibitors—sweet success for diabetic kidney disease? J Am Soc Nephrol. 2017;28(1):7-10. doi:10.1681/asn.2016060650 [PMC free article: PMC5198295] [PubMed: 27539605] [CrossRef]
- 8.
- Marso SP, Daniels GH, Brown-Frandsen K, et al. Liraglutide and cardiovascular outcomes in type 2 diabetes. N Engl J Med. 2016;375(4):311-322. doi:10.1056/NEJMoa1603827 [PMC free article: PMC4985288] [PubMed: 27295427] [CrossRef]
- 9.
- Marso SP, Bain SC, Consoli A, et al. Semaglutide and cardiovascular outcomes in patients with type 2 diabetes. N Engl J Med. 2016;375(19):1834-1844. doi:10.1056/NEJMoa1607141 [PubMed: 27633186] [CrossRef]
- 10.
- Kidney Disease: Improving Global Outcomes Diabetes Work Group. KDIGO 2022 clinical practice guideline for diabetes management in chronic kidney disease. Kidney Int. 2022;102(5S):S1-S127. doi:10.1016/j.kint.2022.06.008 [PubMed: 36272764] [CrossRef]
- 11.
- Parker ED, Lin J, Mahoney T, et al. Economic costs of diabetes in the U.S. in 2022. Diabetes Care. 2024;47(1):26-43. doi:10.2337/dci23-0085 [PubMed: 37909353] [CrossRef]
- 12.
- United States Renal Data System. 2022 USRDS Annual Data Report: Epidemiology of Kidney Disease in the United States. National Institutes of Health, National Institute of Diabetes and Digestive and Kidney Diseases. Accessed September 22, 2023. https://usrds-adr
.niddk.nih.gov/2022 - 13.
- Bjornstad P, Chao LC, Cree-Green M, et al. Youth-onset type 2 diabetes mellitus: an urgent challenge. Nat Rev Nephrol. 2023;19(3):168-184. doi:10.1038/s41581-022-00645-1 [PMC free article: PMC10182876] [PubMed: 36316388] [CrossRef]
- 14.
- Yokoyama H, Okudaira M, Otani T, et al. Higher incidence of diabetic nephropathy in type 2 than in type 1 diabetes in early-onset diabetes in Japan. Kidney Int. 2000;58(1):302-311. doi:10.1046/j.1523-1755.2000.00166.x [PubMed: 10886575] [CrossRef]
- 15.
- Dart AB, Martens PJ, Rigatto C, Brownell MD, Dean HJ, Sellers EA. Earlier onset of complications in youth with type 2 diabetes. Diabetes Care. 2014;37(2):436-443. doi:10.2337/dc13-0954 [PubMed: 24130346] [CrossRef]
- 16.
- TODAY Study Group, Zeitler P, Hirst K, et al. A clinical trial to maintain glycemic control in youth with type 2 diabetes. N Engl J Med. 2012;366(24):2247-2256. doi:10.1056/NEJMoa1109333 [PMC free article: PMC3478667] [PubMed: 22540912] [CrossRef]
- 17.
- American Diabetes Association Professional Practice Committee. 11. Chronic Kidney Disease and Risk Management: Standards of Care in Diabetes-2024. Diabetes Care. 2024;47(Suppl 1):S219-S230. doi:10.2337/dc24-S011 [PMC free article: PMC10725805] [PubMed: 38078574] [CrossRef]
- 18.
- He F, Xia X, Wu XF, Yu XQ, Huang FX. Diabetic retinopathy in predicting diabetic nephropathy in patients with type 2 diabetes and renal disease: a meta-analysis. Diabetologia. 2013;56(3):457-466. doi:10.1007/s00125-012-2796-6 [PubMed: 23232641] [CrossRef]
- 19.
- Tuttle KR, Bakris GL, Bilous RW, et al. Diabetic kidney disease: a report from an ADA Consensus Conference. Diabetes Care. 2014;37(10):2864-2883. doi:10.2337/dc14-1296 [PMC free article: PMC4170131] [PubMed: 25249672] [CrossRef]
- 20.
- Wolf G, Müller N, Mandecka A, Müller UA. Association of diabetic retinopathy and renal function in patients with types 1 and 2 diabetes mellitus. Clin Nephrol. 2007;68(2):81-86. doi:10.5414/cnp68081 [PubMed: 17722706] [CrossRef]
- 21.
- Molitch ME. Management of early diabetic nephropathy. Am J Med. 1997;102(4):392-398. doi:10.1016/s0002-9343(97)00118-6 [PubMed: 9217622] [CrossRef]
- 22.
- Mogensen CE. Prediction of clinical diabetic nephropathy in IDDM patients. Alternatives to microalbuminuria? Diabetes. 1990;39(7):761-767. doi:10.2337/diab.39.7.761 [PubMed: 2191882] [CrossRef]
- 23.
- Ruggenenti P, Porrini EL, Gaspari F, et al. Glomerular hyperfiltration and renal disease progression in type 2 diabetes. Diabetes Care. 2012;35(10):2061-2068. doi:10.2337/dc11-2189 [PMC free article: PMC3447826] [PubMed: 22773704] [CrossRef]
- 24.
- Ficociello LH, Perkins BA, Roshan B, et al. Renal hyperfiltration and the development of microalbuminuria in type 1 diabetes. Diabetes Care. 2009;32(5):889-893. doi:10.2337/dc08-1560 [PMC free article: PMC2671109] [PubMed: 19196883] [CrossRef]
- 25.
- Thomas MC, Moran JL, Harjutsalo V, et al. Hyperfiltration in type 1 diabetes: does it exist and does it matter for nephropathy? Diabetologia. 2012;55(5):1505-1513. doi:10.1007/s00125-012-2485-5 [PubMed: 22322918] [CrossRef]
- 26.
- Moriya T, Tsuchiya A, Okizaki S, Hayashi A, Tanaka K, Shichiri M. Glomerular hyperfiltration and increased glomerular filtration surface are associated with renal function decline in normo- and microalbuminuric type 2 diabetes. Kidney Int. 2012;81(5):486-493. doi:10.1038/ki.2011.404 [PubMed: 22157655] [CrossRef]
- 27.
- Murussi M, Gross JL, Silveiro SP. Glomerular filtration rate changes in normoalbuminuric and microalbuminuric type 2 diabetic patients and normal individuals. A 10-year follow-up. J Diabetes Complications. 2006;20(4):210-215. doi:10.1016/j.jdiacomp.2005.07.002 [PubMed: 16798471] [CrossRef]
- 28.
- Chaiken RL, Eckert-Norton M, Bard M, et al. Hyperfiltration in African-American patients with type 2 diabetes. Cross-sectional and longitudinal data. Diabetes Care. 1998;21(12):2129-2134. doi:10.2337/diacare.21.12.2129 [PubMed: 9839105] [CrossRef]
- 29.
- Brenner BM, Lawler EV, Mackenzie HS. The hyperfiltration theory: a paradigm shift in nephrology. Kidney Int. 1996;49(6):1774-1777. doi:10.1038/ki.1996.265 [PubMed: 8743495] [CrossRef]
- 30.
- Piani F, Melena I, Severn C, et al. Tubular injury in diabetic ketoacidosis: results from the Diabetic Kidney Alarm study. Pediatr Diabetes. 2021;22(7):1031-1039. doi:10.1111/pedi.13259 [PMC free article: PMC8957478] [PubMed: 34435718] [CrossRef]
- 31.
- Melena I, Piani F, Tommerdahl KL, et al. Aminoaciduria and metabolic dysregulation during diabetic ketoacidosis: results from the Diabetic Kidney Alarm (DKA) study. J Diabetes Complications. 2022;36(6):108203. doi:10.1016/j.jdiacomp.2022.108203 [PMC free article: PMC9119939] [PubMed: 35523653] [CrossRef]
- 32.
- Mogensen CE, Christensen CK, Vittinghus E. The stages in diabetic renal disease. With emphasis on the stage of incipient diabetic nephropathy. Diabetes. 1983;32(Suppl 2):64-78. doi:10.2337/diab.32.2.s64 [PubMed: 6400670] [CrossRef]
- 33.
- Martin P, Hampton KK, Walton C, Tindall H, Davies JA. Microproteinuria in type 2 diabetes mellitus from diagnosis. Diabet Med. 1990;7(4):315-318. doi:10.1111/j.1464-5491.1990.tb01396.x [PubMed: 1692521] [CrossRef]
- 34.
- Mogensen C. A complete screening of urinary albumin concentration in an unselected diabetic out-patient clinic population. Diabetic Nephropathy. 1983;2(4):11-18.
- 35.
- de Boer IH, Rue TC, Cleary PA, et al. Long-term renal outcomes of patients with type 1 diabetes mellitus and microalbuminuria: an analysis of the Diabetes Control and Complications Trial/Epidemiology of Diabetes Interventions and Complications cohort. Arch Intern Med. 2011;171(5):412-420. doi:10.1001/archinternmed.2011.16 [PMC free article: PMC3085024] [PubMed: 21403038] [CrossRef]
- 36.
- Hovind P, Tarnow L, Rossing P, et al. Predictors for the development of microalbuminuria and macroalbuminuria in patients with type 1 diabetes: inception cohort study. BMJ. 2004;328(7448):1105. doi:10.1136/bmj.38070.450891.FE [PMC free article: PMC406322] [PubMed: 15096438] [CrossRef]
- 37.
- Viberti GC, Walker JD, Pinto LC. Diabetic nephropathy. In: Alberti KGMM, DeFronzo RA, Keen H, Zimmet P, eds. International Textbook of Diabetes Mellitus. John Wiley & Sons; 1992:1267-1328.
- 38.
- Perkins BA, Ficociello LH, Silva KH, Finkelstein DM, Warram JH, Krolewski AS. Regression of microalbuminuria in type 1 diabetes. N Engl J Med. 2003;348(23):2285-2293. doi:10.1056/NEJMoa021835 [PubMed: 12788992] [CrossRef]
- 39.
- Parving HH, Mauer M, Fioretto P, Rossing P, Ritz E. Diabetic nephropathy. In: Taal MW, Chertow GM, Marsden PA, Skorecki K, Yu ASL, Brenner BM, eds. Brenner & Rector’s The Kidney. 9th ed. Saunders Elsevier; 2011:1411-1443.
- 40.
- Bjornstad P, Maahs DM, Johnson RJ, Rewers M, Snell-Bergeon JK. Estimated insulin sensitivity predicts regression of albuminuria in type 1 diabetes. Diabet Med. 2015;32(2):257-261. doi:10.1111/dme.12572 [PMC free article: PMC4301993] [PubMed: 25303233] [CrossRef]
- 41.
- Bjornstad P, Maahs DM, Duca LM, et al. Estimated insulin sensitivity predicts incident micro- and macrovascular complications in adults with type 1 diabetes over 6 years: the Coronary Artery Calcification in Type 1 Diabetes study. J Diabetes Complications. 2016;30(4):586-590. doi:10.1016/j.jdiacomp.2016.02.011 [PMC free article: PMC4834265] [PubMed: 26936306] [CrossRef]
- 42.
- Mogensen CE, Poulsen PL. Epidemiology of microalbuminuria in diabetes and in the background population. Curr Opin Nephrol Hypertens. 1994;3(3):248-256. doi:10.1097/00041552-199405000-00004 [PubMed: 7922249] [CrossRef]
- 43.
- Jerums G, Panagiotopoulos S, Premaratne E, MacIsaac RJ. Integrating albuminuria and GFR in the assessment of diabetic nephropathy. Nat Rev Nephrol. 2009;5(7):397-406. doi:10.1038/nrneph.2009.91 [PubMed: 19556994] [CrossRef]
- 44.
- TODAY Study Group, Bjornstad P, Drews KL, et al. Long-term complications in youth-onset type 2 diabetes. N Engl J Med. 2021;385(5):416-426. doi:10.1056/NEJMoa2100165 [PMC free article: PMC8697255] [PubMed: 34320286] [CrossRef]
- 45.
- Gall MA, Rossing P, Skøtt P, et al. Prevalence of micro- and macroalbuminuria, arterial hypertension, retinopathy and large vessel disease in European type 2 (non-insulin-dependent) diabetic patients. Diabetologia. 1991;34(9):655-661. doi:10.1007/BF00400995 [PubMed: 1955098] [CrossRef]
- 46.
- Niskanen LK, Penttilã I, Parviainen M, Uusitupa MI. Evolution, risk factors, and prognostic implications of albuminuria in NIDDM. Diabetes Care. 1996;19(5):486-493. doi:10.2337/diacare.19.5.486 [PubMed: 8732714] [CrossRef]
- 47.
- Parving HH, Gall MA, Skøtt P, et al. Prevalence and causes of albuminuria in non-insulin-dependent diabetic patients. Kidney Int. 1992;41(4):758-762. doi:10.1038/ki.1992.118 [PubMed: 1513098] [CrossRef]
- 48.
- Sharma SG, Bomback AS, Radhakrishnan J, et al. The modern spectrum of renal biopsy findings in patients with diabetes. Clin J Am Soc Nephrol. 2013;8(10):1718-1724. doi:10.2215/CJN.02510213 [PMC free article: PMC3789339] [PubMed: 23886566] [CrossRef]
- 49.
- Lindeman RD. Overview: renal physiology and pathophysiology of aging. Am J Kidney Dis. 1990;16(4):275-282. doi:10.1016/s0272-6386(12)80002-3 [PubMed: 2220770] [CrossRef]
- 50.
- Tan JC, Busque S, Workeneh B, et al. Effects of aging on glomerular function and number in living kidney donors. Kidney Int. 2010;78(7):686-692. doi:10.1038/ki.2010.128 [PMC free article: PMC3353650] [PubMed: 20463656] [CrossRef]
- 51.
- Hoang K, Tan JC, Derby G, et al. Determinants of glomerular hypofiltration in aging humans. Kidney Int. 2003;64(4):1417-1424. doi:10.1046/j.1523-1755.2003.00207.x [PubMed: 12969161] [CrossRef]
- 52.
- Palatini P. Glomerular hyperfiltration: a marker of early renal damage in pre-diabetes and pre-hypertension. Nephrol Dial Transplant. 2012;27(5):1708-1714. doi:10.1093/ndt/gfs037 [PubMed: 22431709] [CrossRef]
- 53.
- Hostetter TH, Rennke HG, Brenner BM. The case for intrarenal hypertension in the initiation and progression of diabetic and other glomerulopathies. Am J Med. 1982;72(3):375-380. doi:10.1016/0002-9343(82)90490-9 [PubMed: 7036732] [CrossRef]
- 54.
- Ditzel J, Schwartz M. Abnormally increased glomerular filtration rate in short-term insulin-treated diabetic subjects. Diabetes. 1967;16(4):264-267. doi:10.2337/diab.16.4.264 [PubMed: 6023168] [CrossRef]
- 55.
- Bröchner-Mortensen J. Glomerular filtration rate and extracellular fluid volumes during normoglycemia and moderate hyperglycemia in diabetics. Scand J Clin Lab Invest. 1973;32(4):311-316. doi:10.3109/00365517309084353 [PubMed: 4203766] [CrossRef]
- 56.
- Hostetter TH, Troy JL, Brenner BM. Glomerular hemodynamics in experimental diabetes mellitus. Kidney Int. 1981;19(3):410-415. doi:10.1038/ki.1981.33 [PubMed: 7241881] [CrossRef]
- 57.
- Mogensen CE. Kidney function and glomerular permeability to macromolecules in early juvenile diabetes. Scand J Clin Lab Invest. 1971;28(1):79-90. doi:10.3109/00365517109090666 [PubMed: 5093522] [CrossRef]
- 58.
- Christiansen JS, Gammelgaard J, Frandsen M, Parving HH. Increased kidney size, glomerular filtration rate and renal plasma flow in short-term insulin-dependent diabetics. Diabetologia. 1981;20(4):451-456. doi:10.1007/BF00253406 [PubMed: 7016638] [CrossRef]
- 59.
- Vora JP, Dolben J, Dean JD, et al. Renal hemodynamics in newly presenting non-insulin dependent diabetes mellitus. Kidney Int. 1992;41(4):829-835. doi:10.1038/ki.1992.127 [PubMed: 1513105] [CrossRef]
- 60.
- Cherney DZ, Scholey JW, Miller JA. Insights into the regulation of renal hemodynamic function in diabetic mellitus. Curr Diabetes Rev. 2008;4(4):280-290. doi:10.2174/157339908786241151 [PubMed: 18991597] [CrossRef]
- 61.
- Vervoort G, Veldman B, Berden JH, Smits P, Wetzels JF. Glomerular hyperfiltration in type 1 diabetes mellitus results from primary changes in proximal tubular sodium handling without changes in volume expansion. Eur J Clin Invest. 2005;35(5):330-336. doi:10.1111/j.1365-2362.2005.01497.x [PubMed: 15860045] [CrossRef]
- 62.
- Persson P, Hansell P, Palm F. Tubular reabsorption and diabetes-induced glomerular hyperfiltration. Acta Physiol (Oxf). 2010;200(1):3-10. doi:10.1111/j.1748-1716.2010.02147.x [PMC free article: PMC2919631] [PubMed: 20518753] [CrossRef]
- 63.
- Keller CK, Bergis KH, Fliser D, Ritz E. Renal findings in patients with short-term type 2 diabetes. J Am Soc Nephrol. 1996;7(12):2627-2635. doi:10.1681/ASN.V7122627 [PubMed: 8989741] [CrossRef]
- 64.
- Myers BD, Nelson RG, Williams GW, et al. Glomerular function in Pima Indians with noninsulin-dependent diabetes mellitus of recent onset. J Clin Invest. 1991;88(2):524-530. doi:10.1172/JCI115335 [PMC free article: PMC295379] [PubMed: 1864963] [CrossRef]
- 65.
- Mauer M, Drummond K. The early natural history of nephropathy in type 1 diabetes: I. Study design and baseline characteristics of the study participants. Diabetes. 2002;51(5):1572-1579. doi:10.2337/diabetes.51.5.1572 [PubMed: 11978658] [CrossRef]
- 66.
- Caramori ML, Gross JL, Pecis M, de Azevedo MJ. Glomerular filtration rate, urinary albumin excretion rate, and blood pressure changes in normoalbuminuric normotensive type 1 diabetic patients: an 8-year follow-up study. Diabetes Care. 1999;22(9):1512-1516. doi:10.2337/diacare.22.9.1512 [PubMed: 10480518] [CrossRef]
- 67.
- Jerums G, Premaratne E, Panagiotopoulos S, MacIsaac RJ. The clinical significance of hyperfiltration in diabetes. Diabetologia. 2010;53(10):2093-2104. doi:10.1007/s00125-010-1794-9 [PubMed: 20496053] [CrossRef]
- 68.
- Denic A, Mathew J, Lerman LO, et al. Single-nephron glomerular filtration rate in healthy adults. N Engl J Med. 2017;376(24):2349-2357. doi:10.1056/NEJMoa1614329 [PMC free article: PMC5664219] [PubMed: 28614683] [CrossRef]
- 69.
- Magee GM, Bilous RW, Cardwell CR, Hunter SJ, Kee F, Fogarty DG. Is hyperfiltration associated with the future risk of developing diabetic nephropathy? A meta-analysis. Diabetologia 2009;52(4):691-697. doi:10.1007/s00125-009-1268-0 [PubMed: 19198800] [CrossRef]
- 70.
- Wesson DE. Moving closer to an understanding of the hyperfiltration of type 2 diabetes mellitus. Am J Physiol Regul Integr Comp Physiol. 2006;290(4):R973-R974. doi:10.1152/ajpregu.00853.2005 [PubMed: 16537822] [CrossRef]
- 71.
- Levine DZ, Iacovitti M, Robertson SJ, Mokhtar GA. Modulation of single-nephron GFR in the db/db mouse model of type 2 diabetes mellitus. Am J Physiol Regul Integr Comp Physiol. 2006;290(4):R975-R981. doi:10.1152/ajpregu.00693.2005 [PubMed: 16339386] [CrossRef]
- 72.
- Lervang HH, Jensen S, Brøchner-Mortensen J, Ditzel J. Early glomerular hyperfiltration and the development of late nephropathy in type 1 (insulin-dependent) diabetes mellitus. Diabetologia. 1988;31(10):723-729. doi:10.1007/BF00274773 [PubMed: 3071482] [CrossRef]
- 73.
- Sasson AN, Cherney DZ. Renal hyperfiltration related to diabetes mellitus and obesity in human disease. World J Diabetes. 2012;3(1):1-6. doi:10.4239/wjd.v3.i1.1 [PMC free article: PMC3258534] [PubMed: 22253940] [CrossRef]
- 74.
- Melsom T, Mathisen UD, Ingebretsen OC, et al. Impaired fasting glucose is associated with renal hyperfiltration in the general population. Diabetes Care. 2011;34(7):1546-1551. doi:10.2337/dc11-0235 [PMC free article: PMC3120190] [PubMed: 21593291] [CrossRef]
- 75.
- Mogensen CE, Andersen MJ. Increased kidney size and glomerular filtration rate in untreated juvenile diabetes: normalization by insulin-treatment. Diabetologia. 1975;11(3):221-224. doi:10.1007/BF00422325 [PubMed: 1149954] [CrossRef]
- 76.
- Christiansen JS, Gammelgaard J, Tronier B, Svendsen PA, Parving HH. Kidney function and size in diabetics before and during initial insulin treatment. Kidney Int. 1982;21(5):683-688. doi:10.1038/ki.1982.81 [PubMed: 7050506] [CrossRef]
- 77.
- Schmitz A, Hansen HH, Christensen T. Kidney function in newly diagnosed type 2 (non-insulin-dependent) diabetic patients, before and during treatment. Diabetologia. 1989;32(7):434-439. doi:10.1007/BF00271263 [PubMed: 2806767] [CrossRef]
- 78.
- García Puig J, Mateos Antón F, Grande C, et al. Relation of kidney size to kidney function in early insulin-dependent diabetes. Diabetologia. 1981;21(4):363-367. doi:10.1007/BF00252682 [PubMed: 7286496] [CrossRef]
- 79.
- Vora JP, Dolben J, Williams JD, Peters JR, Owens DR. Impact of initial treatment on renal function in newly-diagnosed type 2 (non-insulin-dependent) diabetes mellitus. Diabetologia. 1993;36(8):734-740. doi:10.1007/BF00401144 [PubMed: 8405740] [CrossRef]
- 80.
- Mogensen CE, Andersen MJ. Increased kidney size and glomerular filtration rate in early juvenile diabetes. Diabetes. 1973;22(9):706-712. doi:10.2337/diab.22.9.706 [PubMed: 4728209] [CrossRef]
- 81.
- Lemley KV, Abdullah I, Myers BD, et al. Evolution of incipient nephropathy in type 2 diabetes mellitus. Kidney Int. 2000;58(3):1228-1237. doi:10.1046/j.1523-1755.2000.00223.x [PubMed: 10972685] [CrossRef]
- 82.
- Lemley KV, Boothroyd DB, Blouch KL, et al. Modeling GFR trajectories in diabetic nephropathy. Am J Physiol Renal Physiol. 2005;289(4):F863-F870. doi:10.1152/ajprenal.00068.2004 [PubMed: 15900022] [CrossRef]
- 83.
- Nelson RG, Knowler WC, Pettitt DJ, Bennett PH. Kidney diseases in diabetes. In: Harris MI, Cowie CC, Stern MP, Boyko EJ, Reiber GE, Bennett PH, eds. Diabetes in America. 2nd ed. National Institutes of Health, NIH Pub No. 95-1468; 1995:349-400.
- 84.
- Christensen CK, Mogensen CE. The course of incipient diabetic nephropathy: studies of albumin excretion and blood pressure. Diabet Med. 1985;2(2):97-102. doi:10.1111/j.1464-5491.1985.tb00608.x [PubMed: 2952409] [CrossRef]
- 85.
- Nelson R, Bennett P, Williams G, et al. Glomerular function in Pima Indians with type 2 (non-insulin-dependent) diabetes. Diabetologia. 1990;33(Suppl):A48. doi:10.1007/BF00401950 [PubMed: 2401399] [CrossRef]
- 86.
- Schmitz A, Christensen T, Møller A, Mogensen CE. Kidney function and cardiovascular risk factors in non-insulin-dependent diabetics (NIDDM) with microalbuminuria. J Intern Med. 1990;228(4):347-352. doi:10.1111/j.1365-2796.1990.tb00244.x [PubMed: 2266344] [CrossRef]
- 87.
- Nelson RG, Beck GJ, Myers BD. Course of glomerular injury in Pima Indians with early diabetic nephropathy. J Am Soc Nephrol. 1993;4(3):306. doi:10.1681/ASN.V43245 [CrossRef]
- 88.
- Pavkov ME, Knowler WC, Lemley KV, Mason CC, Myers BD, Nelson RG. Early renal function decline in type 2 diabetes. Clin J Am Soc Nephrol. 2012;7(1):78-84. doi:10.2215/CJN.07610711 [PMC free article: PMC3265342] [PubMed: 22076874] [CrossRef]
- 89.
- Skupien J, Warram JH, Smiles AM, et al. The early decline in renal function in patients with type 1 diabetes and proteinuria predicts the risk of end-stage renal disease. Kidney Int. 2012;82(5):589-597. doi:10.1038/ki.2012.189 [PMC free article: PMC3425658] [PubMed: 22622493] [CrossRef]
- 90.
- Perkins BA, Ficociello LH, Roshan B, Warram JH, Krolewski AS. In patients with type 1 diabetes and new-onset microalbuminuria the development of advanced chronic kidney disease may not require progression to proteinuria. Kidney Int. 2010;77(1):57-64. doi:10.1038/ki.2009.399 [PMC free article: PMC3725722] [PubMed: 19847154] [CrossRef]
- 91.
- Mauer SM, Steffes MW, Chern M, Brown DM. Mesangial uptake and processing of macromolecules in rats with diabetes mellitus. Lab Invest. 1979;41(5):401-406. [PubMed: 502472]
- 92.
- Viberti GC, Mackintosh D, Bilous RW, Pickup JC, Keen H. Proteinuria in diabetes mellitus: role of spontaneous and experimental variation of glycemia. Kidney Int. 1982;21(5):714-720. doi:10.1038/ki.1982.87 [PubMed: 7050509] [CrossRef]
- 93.
- Kaysen GA, Myers BD, Couser WG, Rabkin R, Felts JM. Mechanisms and consequences of proteinuria. Lab Invest. 1986;54(5):479-498. [PubMed: 3517485]
- 94.
- Brenner BM, Hostetter TH, Humes HD. Glomerular permselectivity: barrier function based on discrimination of molecular size and charge. Am J Physiol. 1978;234(6):F455-F460. doi:10.1152/ajprenal.1978.234.6.F455 [PubMed: 665772] [CrossRef]
- 95.
- Mauer SM, Steffes MW, Ellis EN, Sutherland DE, Brown DM, Goetz FC. Structural-functional relationships in diabetic nephropathy. J Clin Invest. 1984;74(4):1143-1155. doi:10.1172/JCI111523 [PMC free article: PMC425280] [PubMed: 6480821] [CrossRef]
- 96.
- Qian Y, Feldman E, Pennathur S, Kretzler M, Brosius FC, 3rd. From fibrosis to sclerosis: mechanisms of glomerulosclerosis in diabetic nephropathy. Diabetes. 2008;57(6):1439-1445. doi:10.2337/db08-0061 [PMC free article: PMC4239998] [PubMed: 18511444] [CrossRef]
- 97.
- Anderson S, Vora JP. Current concepts of renal hemodynamics in diabetes. J Diabetes Complications. 1995;9(4):304-307. doi:10.1016/1056-8727(95)80028-d [PubMed: 8573753] [CrossRef]
- 98.
- Kanwar YS, Wada J, Sun L, et al. Diabetic nephropathy: mechanisms of renal disease progression. Exp Biol Med (Maywood). 2008;233(1):4-11. doi:10.3181/0705-MR-134 [PubMed: 18156300] [CrossRef]
- 99.
- Gomez DM. Evaluation of renal resistances, with special reference to changes in essential hypertension. J Clin Invest. 1951;30(10):1143-1155. doi:10.1172/JCI102534 [PMC free article: PMC436356] [PubMed: 14888692] [CrossRef]
- 100.
- Bjornstad P, Škrtić M, Lytvyn Y, Maahs DM, Johnson RJ, Cherney DZ. The Gomez’ equations and renal hemodynamic function in kidney disease research. Am J Physiol Renal Physiol. 2016;311(5):F967-F975. doi:10.1152/ajprenal.00415.2016 [PMC free article: PMC6347069] [PubMed: 27605583] [CrossRef]
- 101.
- Fufaa GD, Weil EJ, Lemley KV, et al. Structural predictors of loss of renal function in American Indians with type 2 diabetes. Clin J Am Soc Nephrol. 2016;11(2):254-261. doi:10.2215/CJN.05760515 [PMC free article: PMC4741038] [PubMed: 26792530] [CrossRef]
- 102.
- Steinke JM, Sinaiko AR, Kramer MS, et al. The early natural history of nephropathy in type 1 diabetes: III. Predictors of 5-year urinary albumin excretion rate patterns in initially normoalbuminuric patients. Diabetes. 2005;54(7):2164-2171. doi:10.2337/diabetes.54.7.2164 [PubMed: 15983218] [CrossRef]
- 103.
- Perrin NE, Torbjörnsdotter T, Jaremko GA, Berg UB. Risk markers of future microalbuminuria and hypertension based on clinical and morphological parameters in young type 1 diabetes patients. Pediatr Diabetes. 2010;11(5):305-313. doi:10.1111/j.1399-5448.2009.00595.x [PubMed: 19761528] [CrossRef]
- 104.
- Caramori ML, Parks A, Mauer M. Renal lesions predict progression of diabetic nephropathy in type 1 diabetes. J Am Soc Nephrol. 2013;24(7):1175-1181. doi:10.1681/ASN.2012070739 [PMC free article: PMC3699823] [PubMed: 23687360] [CrossRef]
- 105.
- Fioretto P, Mauer M, Brocco E, et al. Patterns of renal injury in NIDDM patients with microalbuminuria. Diabetologia. 1996;39(12):1569-1576. doi:10.1007/s001250050616 [PubMed: 8960844] [CrossRef]
- 106.
- Østerby R, Gall MA, Schmitz A, Nielsen FS, Nyberg G, Parving HH. Glomerular structure and function in proteinuric type 2 (non-insulin-dependent) diabetic patients. Diabetologia. 1993;36(10):1064-1070. doi:10.1007/BF02374500 [PubMed: 8243856] [CrossRef]
- 107.
- Nosadini R, Velussi M, Brocco E, et al. Course of renal function in type 2 diabetic patients with abnormalities of albumin excretion rate. Diabetes. 2000;49(3):476-484. doi:10.2337/diabetes.49.3.476 [PubMed: 10868971] [CrossRef]
- 108.
- Moriya T, Suzuki Y, Inomata S, Iwano M, Kanauchi M, Haneda M. Renal histological heterogeneity and functional progress in normoalbuminuric and microalbuminuric Japanese patients with type 2 diabetes. BMJ Open Diabetes Res Care. 2014;2(1):e000029. doi:10.1136/bmjdrc-2014-000029 [PMC free article: PMC4212561] [PubMed: 25452869] [CrossRef]
- 109.
- Dane MJ, Khairoun M, Lee DH, et al. Association of kidney function with changes in the endothelial surface layer. Clin J Am Soc Nephrol. 2014;9(4):698-704. doi:10.2215/CJN.08160813 [PMC free article: PMC3974363] [PubMed: 24458084] [CrossRef]
- 110.
- Satchell S. The role of the glomerular endothelium in albumin handling. Nat Rev Nephrol. 2013;9(12):717-725. doi:10.1038/nrneph.2013.197 [PubMed: 24080802] [CrossRef]
- 111.
- Garsen M, Rops AL, Rabelink TJ, Berden JH, van der Vlag J. The role of heparanase and the endothelial glycocalyx in the development of proteinuria. Nephrol Dial Transplant. 2014;29(1):49-55. doi:10.1093/ndt/gft410 [PubMed: 24166469] [CrossRef]
- 112.
- Zhang C, Meng Y, Liu Q, et al. Injury to the endothelial surface layer induces glomerular hyperfiltration rats with early-stage diabetes. J Diabetes Res. 2014;2014:953740. doi:10.1155/2014/953740 [PMC free article: PMC4000657] [PubMed: 24812636] [CrossRef]
- 113.
- Satchell SC, Braet F. Glomerular endothelial cell fenestrations: an integral component of the glomerular filtration barrier. Am J Physiol Renal Physiol. 2009;296(5):F947-F956. doi:10.1152/ajprenal.90601.2008 [PMC free article: PMC2681366] [PubMed: 19129259] [CrossRef]
- 114.
- Ballermann BJ. Contribution of the endothelium to the glomerular permselectivity barrier in health and disease. Nephron Physiol. 2007;106(2):19-25. doi:10.1159/000101796 [PubMed: 17570944] [CrossRef]
- 115.
- Weil EJ, Lemley KV, Mason CC, et al. Podocyte detachment and reduced glomerular capillary endothelial fenestration promote kidney disease in type 2 diabetic nephropathy. Kidney Int. 2012;82(9):1010-1017. doi:10.1038/ki.2012.234 [PMC free article: PMC3472108] [PubMed: 22718189] [CrossRef]
- 116.
- Tervaert TW, Mooyaart AL, Amann K, et al. Pathologic classification of diabetic nephropathy. J Am Soc Nephrol. 2010;21(4):556-563. doi:10.1681/ASN.2010010010 [PubMed: 20167701] [CrossRef]
- 117.
- White KE, Bilous RW. Type 2 diabetic patients with nephropathy show structural-functional relationships that are similar to type 1 disease. J Am Soc Nephrol. 2000;11(9):1667-1673. doi:10.1681/ASN.V1191667 [PubMed: 10966491] [CrossRef]
- 118.
- Caramori ML, Kim Y, Huang C, et al. Cellular basis of diabetic nephropathy: 1. Study design and renal structural-functional relationships in patients with long-standing type 1 diabetes. Diabetes. 2002;51(2):506-513. doi:10.2337/diabetes.51.2.506 [PubMed: 11812762] [CrossRef]
- 119.
- Poole G. Intercapillary glomerulosclerosis and the Kimmelstiel-Wilson syndrome. Postgrad Med J. 1953;29(329):137-146. doi:10.1136/pgmj.29.329.137 [PMC free article: PMC2500335] [PubMed: 13055534] [CrossRef]
- 120.
- Najafian B, Alpers CE, Fogo AB. Pathology of human diabetic nephropathy. Contrib Nephrol. 2011;170:36-47. doi:10.1159/000324942 [PubMed: 21659756] [CrossRef]
- 121.
- Schwartz MM, Lewis EJ, Leonard-Martin T, Lewis JB, Batlle D. Renal pathology patterns in type II diabetes mellitus: relationship with retinopathy. The Collaborative Study Group. Nephrol Dial Transplant. 1998;13(10):2547-2552. doi:10.1093/ndt/13.10.2547 [PubMed: 9794557] [CrossRef]
- 122.
- Østerby R, Parving HH, Hommel E, Jørgensen HE, Løkkegaard H. Glomerular structure and function in diabetic nephropathy. Early to advanced stages. Diabetes. 1990;39(9):1057-1063. doi:10.2337/diab.39.9.1057 [PubMed: 2384188] [CrossRef]
- 123.
- Salmon AH, Toma I, Sipos A, et al. Evidence for restriction of fluid and solute movement across the glomerular capillary wall by the subpodocyte space. Am J Physiol Renal Physiol. 2007;293(6):F1777-F1786. doi:10.1152/ajprenal.00187.2007 [PubMed: 17804486] [CrossRef]
- 124.
- Kriz W. Progressive renal failure—inability of podocytes to replicate and the consequences for development of glomerulosclerosis. Nephrol Dial Transplant. 1996;11(9):1738-1742. doi:10.1093/ndt/11.9.1738 [PubMed: 8918614] [CrossRef]
- 125.
- Becker JU, Hoerning A, Schmid KW, Hoyer PF. Immigrating progenitor cells contribute to human podocyte turnover. Kidney Int. 2007;72(12):1468-1473. doi:10.1038/sj.ki.5002524 [PubMed: 17898701] [CrossRef]
- 126.
- Ohse T, Pippin JW, Chang AM, et al. The enigmatic parietal epithelial cell is finally getting noticed: a review. Kidney Int. 2009;76(12):1225-1238. doi:10.1038/ki.2009.386 [PMC free article: PMC3708665] [PubMed: 19847153] [CrossRef]
- 127.
- Shankland SJ, Wolf G. Cell cycle regulatory proteins in renal disease: role in hypertrophy, proliferation, and apoptosis. Am J Physiol Renal Physiol. 2000;278(4):F515-F529. doi:10.1152/ajprenal.2000.278.4.F515 [PubMed: 10751212] [CrossRef]
- 128.
- Pagtalunan ME, Miller PL, Jumping-Eagle S, et al. Podocyte loss and progressive glomerular injury in type II diabetes. J Clin Invest. 1997;99(2):342-348. doi:10.1172/JCI119163 [PMC free article: PMC507802] [PubMed: 9006003] [CrossRef]
- 129.
- Sun YBY, Qu X, Zhang X, Caruana G, Bertram JF, Li J. Glomerular endothelial cell injury and damage precedes that of podocytes in adriamycin-induced nephropathy. PLoS One. 2013;8(1):e55027 doi:10.1371/journal.pone.0055027 [PMC free article: PMC3554670] [PubMed: 23359116] [CrossRef]
- 130.
- Nakagawa T, Sato W, Glushakova O, et al. Diabetic endothelial nitric oxide synthase knockout mice develop advanced diabetic nephropathy. J Am Soc Nephrol. 2007;18(2):539-550. doi:10.1681/ASN.2006050459 [PubMed: 17202420] [CrossRef]
- 131.
- Yuen DA, Stead BE, Zhang Y, et al. eNOS deficiency predisposes podocytes to injury in diabetes. J Am Soc Nephrol. 2012;23(11):1810-1823. doi:10.1681/ASN.2011121170 [PMC free article: PMC3482727] [PubMed: 22997257] [CrossRef]
- 132.
- Dane MJ, van den Berg BM, Avramut MC, et al. Glomerular endothelial surface layer acts as a barrier against albumin filtration. Am J Pathol. 2013;182(5):1532-1540. doi:10.1016/j.ajpath.2013.01.049 [PubMed: 23518410] [CrossRef]
- 133.
- Morigi M, Buelli S, Angioletti S, et al. In response to protein load podocytes reorganize cytoskeleton and modulate endothelin-1 gene: implication for permselective dysfunction of chronic nephropathies. Am J Pathol. 2005;166(5):1309-1320. doi:10.1016/S0002-9440(10)62350-4 [PMC free article: PMC1606387] [PubMed: 15855633] [CrossRef]
- 134.
- Fioretto P, Mauer M. Histopathology of diabetic nephropathy. Semin Nephrol. 2007;27(2):195-207. doi:10.1016/j.semnephrol.2007.01.012 [PMC free article: PMC2746982] [PubMed: 17418688] [CrossRef]
- 135.
- Torbjörnsdotter TB, Perrin NE, Jaremko GA, Berg UB. Widening of foot processes in normoalbuminuric adolescents with type 1 diabetes. Pediatr Nephrol. 2005;20(6):750-758. doi:10.1007/s00467-005-1829-5 [PubMed: 15827743] [CrossRef]
- 136.
- Dalla Vestra M, Masiero A, Roiter AM, Saller A, Crepaldi G, Fioretto P. Is podocyte injury relevant in diabetic nephropathy? Studies in patients with type 2 diabetes. Diabetes. 2003;52(4):1031-1035. doi:10.2337/diabetes.52.4.1031 [PubMed: 12663476] [CrossRef]
- 137.
- Nakamura T, Ushiyama C, Suzuki S, et al. Urinary excretion of podocytes in patients with diabetic nephropathy. Nephrol Dial Transplant. 2000;15(9):1379-1383. doi:10.1093/ndt/15.9.1379 [PubMed: 10978394] [CrossRef]
- 138.
- Siu B, Saha J, Smoyer WE, Sullivan KA, Brosius FC, 3rd. Reduction in podocyte density as a pathologic feature in early diabetic nephropathy in rodents: prevention by lipoic acid treatment. BMC Nephrol. 2006;7:6. doi:10.1186/1471-2369-7-6 [PMC free article: PMC1435876] [PubMed: 16539708] [CrossRef]
- 139.
- Ronconi E, Sagrinati C, Angelotti ML, et al. Regeneration of glomerular podocytes by human renal progenitors. J Am Soc Nephrol. 2009;20(2):322-332. doi:10.1681/ASN.2008070709 [PMC free article: PMC2637058] [PubMed: 19092120] [CrossRef]
- 140.
- Shankland SJ, Anders HJ, Romagnani P. Glomerular parietal epithelial cells in kidney physiology, pathology, and repair. Curr Opin Nephrol Hypertens. 2013;22(3):302-309. doi:10.1097/MNH.0b013e32835fefd4 [PubMed: 23518463] [CrossRef]
- 141.
- Bohle A, Wehrmann M, Bogenschutz O, Batz C, Muller CA, Muller GA. The pathogenesis of chronic renal failure in diabetic nephropathy. Investigation of 488 cases of diabetic glomerulosclerosis. Pathol Res Pract. 1991;187(2-3):251-259. doi:10.1016/s0344-0338(11)80780-6 [PubMed: 2068008] [CrossRef]
- 142.
- Najafian B, Mauer M. Progression of diabetic nephropathy in type 1 diabetic patients. Diabetes Res Clin Pract. 2009;83(1):1-8. doi:10.1016/j.diabres.2008.08.024 [PubMed: 19070384] [CrossRef]
- 143.
- Stout LC, Kumar S, Whorton EB. Insudative lesions—their pathogenesis and association with glomerular obsolescence in diabetes: a dynamic hypothesis based on single views of advancing human diabetic nephropathy. Hum Pathol. 1994;25(11):1213-1227. doi:10.1016/0046-8177(94)90039-6 [PubMed: 7959667] [CrossRef]
- 144.
- Mauer SM, Lane P, Zhu D, Fioretto P, Steffes MW. Renal structure and function in insulin-dependent diabetes mellitus in man. J Hypertens Suppl. 1992;10(1):S17-S20. doi:10.1097/00004872-199204001-00004 [PubMed: 1619499] [CrossRef]
- 145.
- Horsfield GI, Lannigan R. Exudative lesions in diabetes mellitus. J Clin Pathol. 1965;18(1):47-53. doi:10.1136/jcp.18.1.47 [PMC free article: PMC472832] [PubMed: 14247704] [CrossRef]
- 146.
- Alsaad KO, Herzenberg AM. Distinguishing diabetic nephropathy from other causes of glomerulosclerosis: an update. J Clin Pathol. 2007;60(1):18-26. doi:10.1136/jcp.2005.035592 [PMC free article: PMC1860608] [PubMed: 17213346] [CrossRef]
- 147.
- Najafian B, Crosson JT, Kim Y, Mauer M. Glomerulotubular junction abnormalities are associated with proteinuria in type 1 diabetes. J Am Soc Nephrol. 2006;17(4 Suppl 2):S53-S60. doi:10.1681/ASN.2005121342 [PubMed: 16565248] [CrossRef]
- 148.
- Garg AX, Kiberd BA, Clark WF, Haynes RB, Clase CM. Albuminuria and renal insufficiency prevalence guides population screening: results from the NHANES III. Kidney Int. 2002;61(6):2165-2175. doi:10.1046/j.1523-1755.2002.00356.x [PubMed: 12028457] [CrossRef]
- 149.
- Kramer HJ, Nguyen QD, Curhan G, Hsu CY. Renal insufficiency in the absence of albuminuria and retinopathy among adults with type 2 diabetes mellitus. JAMA. 2003;289(24):3273-3277. doi:10.1001/jama.289.24.3273 [PubMed: 12824208] [CrossRef]
- 150.
- MacIsaac RJ, Tsalamandris C, Panagiotopoulos S, Smith TJ, McNeil KJ, Jerums G. Nonalbuminuric renal insufficiency in type 2 diabetes. Diabetes Care. 2004;27(1):195-200. doi:10.2337/diacare.27.1.195 [PubMed: 14693989] [CrossRef]
- 151.
- Caramori ML, Fioretto P, Mauer M. Low glomerular filtration rate in normoalbuminuric type 1 diabetic patients: an indicator of more advanced glomerular lesions. Diabetes. 2003;52(4):1036-1040. doi:10.2337/diabetes.52.4.1036 [PubMed: 12663477] [CrossRef]
- 152.
- Rigalleau V, Lasseur C, Raffaitin C, et al. Normoalbuminuric renal-insufficient diabetic patients: a lower-risk group. Diabetes Care. 2007;30(8):2034-2039. doi:10.2337/dc07-0140 [PubMed: 17485574] [CrossRef]
- 153.
- Kramer CK, Leitão CB, Pinto LC, Silveiro SP, Gross JL, Canani LH. Clinical and laboratory profile of patients with type 2 diabetes with low glomerular filtration rate and normoalbuminuria. Diabetes Care. 2007;30(8):1998-2000. doi:10.2337/dc07-0387 [PubMed: 17468344] [CrossRef]
- 154.
- Rychlik I, Fliser D, Ritz E. Non-diabetic renal disease in type 2 diabetes mellitus. In: Ritz E, Rychlik I, eds. Nephropathy in Type 2 Diabetes. Oxford University Press; 1999:7-88.
- 155.
- MacIsaac RJ, Panagiotopoulos S, McNeil KJ, et al. Is nonalbuminuric renal insufficiency in type 2 diabetes related to an increase in intrarenal vascular disease? Diabetes Care. 2006;29(7):1560-1566. doi:10.2337/dc05-1788 [PubMed: 16801579] [CrossRef]
- 156.
- Pavkov ME, Mason CC, Bennett PH, Curtis JM, Knowler WC, Nelson RG. Change in the distribution of albuminuria according to estimated glomerular filtration rate in Pima Indians with type 2 diabetes. Diabetes Care. 2009;32(10):1845-1850. doi:10.2337/dc08-2325 [PMC free article: PMC2752932] [PubMed: 19592626] [CrossRef]
- 157.
- Bolignano D, Lacquaniti A, Coppolino G, et al. Neutrophil gelatinase-associated lipocalin (NGAL) and progression of chronic kidney disease. Clin J Am Soc Nephrol. 2009;4(2):337-344. doi:10.2215/CJN.03530708 [PMC free article: PMC2637601] [PubMed: 19176795] [CrossRef]
- 158.
- Nielsen SE, Sugaya T, Hovind P, Baba T, Parving HH, Rossing P. Urinary liver-type fatty acid-binding protein predicts progression to nephropathy in type 1 diabetic patients. Diabetes Care. 2010;33(6):1320-1324. doi:10.2337/dc09-2242 [PMC free article: PMC2875447] [PubMed: 20185732] [CrossRef]
- 159.
- Nielsen SE, Andersen S, Zdunek D, Hess G, Parving HH, Rossing P. Tubular markers do not predict the decline in glomerular filtration rate in type 1 diabetic patients with overt nephropathy. Kidney Int. 2011;79(10):1113-1118. doi:10.1038/ki.2010.554 [PubMed: 21270761] [CrossRef]
- 160.
- von Eynatten M, Baumann M, Heemann U, et al. Urinary L-FABP and anaemia: distinct roles of urinary markers in type 2 diabetes. Eur J Clin Invest. 2010;40(2):95-102. doi:10.1111/j.1365-2362.2009.02220.x [PubMed: 19912308] [CrossRef]
- 161.
- Nielsen SE, Hansen HP, Jensen BR, Parving HH, Rossing P. Urinary neutrophil gelatinase-associated lipocalin and progression of diabetic nephropathy in type 1 diabetic patients in a four-year follow-up study. Nephron Clin Pract. 2011;118(2):c130-135. doi:10.1159/000320615 [PubMed: 21160226] [CrossRef]
- 162.
- Vaidya VS, Niewczas MA, Ficociello LH, et al. Regression of microalbuminuria in type 1 diabetes is associated with lower levels of urinary tubular injury biomarkers, kidney injury molecule-1, and N-acetyl-β-D-glucosaminidase. Kidney Int. 2011;79(4):464-470. doi:10.1038/ki.2010.404 [PMC free article: PMC3033751] [PubMed: 20980978] [CrossRef]
- 163.
- Nielsen SE, Reinhard H, Zdunek D, et al. Tubular markers are associated with decline in kidney function in proteinuric type 2 diabetic patients. Diabetes Res Clin Pract. 2012;97(1):71-76. doi:10.1016/j.diabres.2012.02.007 [PubMed: 22402306] [CrossRef]
- 164.
- Kim SS, Song SH, Kim IJ, et al. Clinical implication of urinary tubular markers in the early stage of nephropathy with type 2 diabetic patients. Diabetes Res Clin Pract. 2012;97(2):251-257. doi:10.1016/j.diabres.2012.02.019 [PubMed: 22440044] [CrossRef]
- 165.
- Fu WJ, Li BL, Wang SB, et al. Changes of the tubular markers in type 2 diabetes mellitus with glomerular hyperfiltration. Diabetes Res Clin Pract. 2012;95(1):105-109. doi:10.1016/j.diabres.2011.09.031 [PubMed: 22015481] [CrossRef]
- 166.
- Conway BR, Manoharan D, Manoharan D, et al. Measuring urinary tubular biomarkers in type 2 diabetes does not add prognostic value beyond established risk factors. Kidney Int. 2012;82(7):812-818. doi:10.1038/ki.2012.218 [PubMed: 22718188] [CrossRef]
- 167.
- Liu KD, Yang W, Anderson AH, et al. Urine neutrophil gelatinase-associated lipocalin levels do not improve risk prediction of progressive chronic kidney disease. Kidney Int. 2013;83(5):909-914. doi:10.1038/ki.2012.458 [PMC free article: PMC3642209] [PubMed: 23344473] [CrossRef]
- 168.
- Araki S, Haneda M, Koya D, et al. Predictive effects of urinary liver-type fatty acid-binding protein for deteriorating renal function and incidence of cardiovascular disease in type 2 diabetic patients without advanced nephropathy. Diabetes Care. 2013;36(5):1248-1253. doi:10.2337/dc12-1298 [PMC free article: PMC3631864] [PubMed: 23223350] [CrossRef]
- 169.
- O’Seaghdha CM, Hwang SJ, Larson MG, Meigs JB, Vasan RS, Fox CS. Analysis of a urinary biomarker panel for incident kidney disease and clinical outcomes. J Am Soc Nephrol. 2013;24(11):1880-1888. doi:10.1681/ASN.2013010019 [PMC free article: PMC3810081] [PubMed: 23990678] [CrossRef]
- 170.
- Chou KM, Lee CC, Chen CH, Sun CY. Clinical value of NGAL, L-FABP and albuminuria in predicting GFR decline in type 2 diabetes mellitus patients. PLoS One. 2013;8(1):e54863. doi:10.1371/journal.pone.0054863 [PMC free article: PMC3551928] [PubMed: 23349979] [CrossRef]
- 171.
- Panduru NM, Forsblom C, Saraheimo M, et al. Urinary liver-type fatty acid-binding protein and progression of diabetic nephropathy in type 1 diabetes. Diabetes Care. 2013;36(7):2077-2083. doi:10.2337/dc12-1868 [PMC free article: PMC3687279] [PubMed: 23378622] [CrossRef]
- 172.
- Kamijo-Ikemori A, Sugaya T, Yasuda T, et al. Clinical significance of urinary liver-type fatty acid-binding protein in diabetic nephropathy of type 2 diabetic patients. Diabetes Care. 2011;34(3):691-696. doi:10.2337/dc10-1392 [PMC free article: PMC3041209] [PubMed: 21273494] [CrossRef]
- 173.
- Fufaa GD, Weil EJ, Nelson RG, et al. Association of urinary KIM-1, L-FABP, NAG and NGAL with incident end-stage renal disease and mortality in American Indians with type 2 diabetes mellitus. Diabetologia. 2015;58(1):188-198. doi:10.1007/s00125-014-3389-3 [PMC free article: PMC4258130] [PubMed: 25316431] [CrossRef]
- 174.
- Aksun SA, Ozmen D, Ozmen B, et al. Beta microglobulin and cystatin C in type 2 diabetes: assessment of diabetic nephropathy. Exp Clin Endocrinol Diabetes. 2004;112(4):195-200. doi:10.1055/s-2004-817933 [PubMed: 15127324] [CrossRef]
- 175.
- Viberti GC, Keen H, Mackintosh D. Beta 2-microglobulinaemia: a sensitive index of diminishing renal function in diabetics. Br Med J (Clin Res Ed). 1981;282(6258):95-98. doi:10.1136/bmj.282.6258.95 [PMC free article: PMC1503840] [PubMed: 6161671] [CrossRef]
- 176.
- Astor BC, Shafi T, Hoogeveen RC, et al. Novel markers of kidney function as predictors of ESRD, cardiovascular disease, and mortality in the general population. Am J Kidney Dis. 2012;59(5):653-662. doi:10.1053/j.ajkd.2011.11.042 [PMC free article: PMC3880682] [PubMed: 22305758] [CrossRef]
- 177.
- Miyazawa I, Araki S, Obata T, et al. Association between serum soluble TNFα receptors and renal dysfunction in type 2 diabetic patients without proteinuria. Diabetes Res Clin Pract. 2011;92(2):174-180. doi:10.1016/j.diabres.2011.01.008 [PubMed: 21288590] [CrossRef]
- 178.
- Lopes-Virella MF, Baker NL, Hunt KJ, et al. Baseline markers of inflammation are associated with progression to macroalbuminuria in type 1 diabetic subjects. Diabetes Care. 2013;36(8):2317-2323. doi:10.2337/dc12-2521 [PMC free article: PMC3714479] [PubMed: 23514730] [CrossRef]
- 179.
- Niewczas MA, Ficociello LH, Johnson AC, et al. Serum concentrations of markers of TNFalpha and Fas-mediated pathways and renal function in nonproteinuric patients with type 1 diabetes. Clin J Am Soc Nephrol. 2009;4(1):62-70. doi:10.2215/CJN.03010608 [PMC free article: PMC2615709] [PubMed: 19073786] [CrossRef]
- 180.
- Niewczas MA, Gohda T, Skupien J, et al. Circulating TNF receptors 1 and 2 predict ESRD in type 2 diabetes. J Am Soc Nephrol. 2012;23(3):507-515. doi:10.1681/ASN.2011060627 [PMC free article: PMC3294310] [PubMed: 22266663] [CrossRef]
- 181.
- Gohda T, Niewczas MA, Ficociello LH, et al. Circulating TNF receptors 1 and 2 predict stage 3 CKD in type 1 diabetes. J Am Soc Nephrol. 2012;23(3):516-524. doi:10.1681/ASN.2011060628 [PMC free article: PMC3294299] [PubMed: 22266664] [CrossRef]
- 182.
- Forsblom C, Moran J, Harjutsalo V, et al. Added value of soluble tumor necrosis factor-α receptor 1 as a biomarker of ESRD risk in patients with type 1 diabetes. Diabetes Care. 2014;37(8):2334-2342. doi:10.2337/dc14-0225 [PubMed: 24879837] [CrossRef]
- 183.
- Saulnier PJ, Gand E, Ragot S, et al. Association of serum concentration of TNFR1 with all-cause mortality in patients with type 2 diabetes and chronic kidney disease: follow-up of the SURDIAGENE Cohort. Diabetes Care. 2014;37(5):1425-1431. doi:10.2337/dc13-2580 [PubMed: 24623026] [CrossRef]
- 184.
- Pavkov ME, Nelson RG, Knowler WC, Cheng Y, Krolewski AS, Niewczas MA. Elevation of circulating TNF receptors 1 and 2 increases the risk of end-stage renal disease in American Indians with type 2 diabetes. Kidney Int. 2015;87(4):812-819. doi:10.1038/ki.2014.330 [PMC free article: PMC4382420] [PubMed: 25272234] [CrossRef]
- 185.
- Niewczas MA, Pavkov ME, Skupien J, et al. A signature of circulating inflammatory proteins and development of end-stage renal disease in diabetes. Nat Med. 2019;25(5):805-813. doi:10.1038/s41591-019-0415-5 [PMC free article: PMC6508971] [PubMed: 31011203] [CrossRef]
- 186.
- Yao Y, Rabodzey A, Dewey CF, Jr. Glycocalyx modulates the motility and proliferative response of vascular endothelium to fluid shear stress. Am J Physiol Heart Circ Physiol. 2007;293(2):H1023-H1030. doi:10.1152/ajpheart.00162.2007 [PubMed: 17468337] [CrossRef]
- 187.
- Jeansson M, Björck K, Tenstad O, Haraldsson B. Adriamycin alters glomerular endothelium to induce proteinuria. J Am Soc Nephrol. 2009;20(1):114-122. doi:10.1681/ASN.2007111205 [PMC free article: PMC2615716] [PubMed: 19073829] [CrossRef]
- 188.
- Wahl P, Deppermann D, Hasslacher C. Biochemistry of glomerular basement membrane of the normal and diabetic human. Kidney Int. 1982;21(5):744-749. doi:10.1038/ki.1982.92 [PubMed: 7109461] [CrossRef]
- 189.
- Vernier RL, Steffes MW, Sisson-Ross S, Mauer SM. Heparan sulfate proteoglycan in the glomerular basement membrane in type 1 diabetes mellitus. Kidney Int. 1992;41(4):1070-1080. doi:10.1038/ki.1992.163 [PubMed: 1513088] [CrossRef]
- 190.
- Alfino PA, Neugarten J, Schacht RG, Dworkin LD, Baldwin DS. Glomerular size-selective barrier dysfunction in nephrotoxic serum nephritis. Kidney Int. 1988;34(2):151-155. doi:10.1038/ki.1988.160 [PubMed: 2460659] [CrossRef]
- 191.
- Andersson M, Nilsson U, Hjalmarsson C, Haraldsson B, Nyström JS. Mild renal ischemia-reperfusion reduces charge and size selectivity of the glomerular barrier. Am J Physiol Renal Physiol. 2007;292(6):F1802-F1809. doi:10.1152/ajprenal.00152.2006 [PubMed: 17376766] [CrossRef]
- 192.
- Bennett CM, Glassock RJ, Chang RL, et al. Permselectivity of the glomerular capillary wall. Studies of experimental glomerulonephritis in the rat using dextran sulfate. J Clin Invest 1976;57(5):1287-1294. doi:10.1172/JCI108396 [PMC free article: PMC436781] [PubMed: 1262472] [CrossRef]
- 193.
- Blouch K, Deen WM, Fauvel JP, Bialek J, Derby G, Myers BD. Molecular configuration and glomerular size selectivity in healthy and nephrotic humans. Am J Physiol. 1997;273(3 Pt 2):F430-F437. doi:10.1152/ajprenal.1997.273.3.F430 [PubMed: 9321916] [CrossRef]
- 194.
- Fox JG, Quin JD, Paterson KR, O’Reilly DS, Smith MP, Boulton-Jones JM. Glomerular charge selectivity in type 1 (insulin-dependent) diabetes mellitus. Diabet Med. 1995;12(5):387-391. doi:10.1111/j.1464-5491.1995.tb00500.x [PubMed: 7648799] [CrossRef]
- 195.
- Groggel GC, Stevenson J, Hovingh P, Linker A, Border WA. Changes in heparan sulfate correlate with increased glomerular permeability. Kidney Int. 1988;33(2):517-523. doi:10.1038/ki.1988.28 [PubMed: 2452273] [CrossRef]
- 196.
- Guasch A, Myers BD. Determinants of glomerular hypofiltration in nephrotic patients with minimal change nephropathy. J Am Soc Nephrol. 1994;4(8):1571-1581. doi:10.1681/ASN.V481571 [PubMed: 8025231] [CrossRef]
- 197.
- Myers BD, Winetz JA, Chui F, Michaels AS. Mechanisms of proteinuria in diabetic nephropathy: a study of glomerular barrier function. Kidney Int. 1982;21(4):633-641. doi:10.1038/ki.1982.71 [PubMed: 6178872] [CrossRef]
- 198.
- Oliver JD, 3rd, Anderson S, Troy JL, Brenner BM, Deen WH. Determination of glomerular size-selectivity in the normal rat with Ficoll. J Am Soc Nephrol. 1992;3(2):214-228. doi:10.1681/ASN.V32214 [PubMed: 1382654] [CrossRef]
- 199.
- Olson JL, Hostetter TH, Rennke HG, Brenner BM, Venkatachalam MA. Altered glomerular permselectivity and progressive sclerosis following extreme ablation of renal mass. Kidney Int. 1982;22(2):112-126. doi:10.1038/ki.1982.143 [PubMed: 6182335] [CrossRef]
- 200.
- Olson JL, Rennke HG, Venkatachalam MA. Alterations in the charge and size selectivity barrier of the glomerular filter in aminonucleoside nephrosis in rats. Lab Invest. 1981;44(3):271-279. [PubMed: 7464051]
- 201.
- Ruggenenti P, Mosconi L, Sangalli F, et al. Glomerular size-selective dysfunction in NIDDM is not ameliorated by ACE inhibition or by calcium channel blockade. Kidney Int. 1999;55(3):984-994. doi:10.1046/j.1523-1755.1999.055003984.x [PubMed: 10027935] [CrossRef]
- 202.
- Scandling JD, Black VM, Deen WM, Myers BD. Glomerular permselectivity in healthy and nephrotic humans. Adv Nephrol Necker Hosp. 1992;21:159-176. [PubMed: 1566645]
- 203.
- Scandling JD, Myers BD. Glomerular size-selectivity and microalbuminuria in early diabetic glomerular disease. Kidney Int. 1992;41(4):840-846. doi:10.1038/ki.1992.129 [PubMed: 1381005] [CrossRef]
- 204.
- Haraldsson B, Sörensson J. Why do we not all have proteinuria? An update of our current understanding of the glomerular barrier. News Physiol Sci 2004;19:7-10. doi:10.1152/nips.01461.2003 [PubMed: 14739395] [CrossRef]
- 205.
- Singh A, Satchell SC, Neal CR, McKenzie EA, Tooke JE, Mathieson PW. Glomerular endothelial glycocalyx constitutes a barrier to protein permeability. J Am Soc Nephrol. 2007;18(11):2885-2893. doi:10.1681/ASN.2007010119 [PubMed: 17942961] [CrossRef]
- 206.
- Singh A, Fridén V, Dasgupta I, et al. High glucose causes dysfunction of the human glomerular endothelial glycocalyx. Am J Physiol Renal Physiol. 2011;300(1):F40-F48. doi:10.1152/ajprenal.00103.2010 [PMC free article: PMC3023224] [PubMed: 20980411] [CrossRef]
- 207.
- Lemley KV, Blouch K, Abdullah I, et al. Glomerular permselectivity at the onset of nephropathy in type 2 diabetes mellitus. J Am Soc Nephrol. 2000;11(11):2095-2105. doi:10.1681/ASN.V11112095 [PubMed: 11053486] [CrossRef]
- 208.
- Deckert T, Kofoed-Enevoldsen A, Vidal P, Nørgaard K, Andreasen HB, Feldt-Rasmussen B. Size-and charge selectivity of glomerular filtration in type 1 (insulin-dependent) diabetic patients with and without albuminuria. Diabetologia. 1993;36(3):244-251. doi:10.1007/BF00399958 [PubMed: 8462774] [CrossRef]
- 209.
- Friedman S, Jones HW, 3rd, Golbetz HV, Lee JA, Little HL, Myers BD. Mechanisms of proteinuria in diabetic nephropathy. II. A study of the size-selective glomerular filtration barrier. Diabetes. 1983;32(Suppl 2):40-46. doi:10.2337/diab.32.2.s40 [PubMed: 6086024] [CrossRef]
- 210.
- Myers BD, Nelson RG, Bennett PH, Mitch WE. Diabetic Renal Disease Study: glomerular function at onset of nephropathy in NIDDM (Abstract). J Am Soc Nephrol. 1991;2:A295
- 211.
- Tomlanovich S, Deen WM, Jones HW, 3rd, Schwartz HC, Myers BD. Functional nature of glomerular injury in progressive diabetic glomerulopathy. Diabetes. 1987;36(5):556-565. doi:10.2337/diab.36.5.556 [PubMed: 2436961] [CrossRef]
- 212.
- Pavenstädt H, Kriz W, Kretzler M. Cell biology of the glomerular podocyte. Physiol Rev. 2003;83(1):253-307. doi:10.1152/physrev.00020.2002 [PubMed: 12506131] [CrossRef]
- 213.
- Peti-Peterdi J, Burford JL, Hackl MJ. The first decade of using multiphoton microscopy for high-power kidney imaging. Am J Physiol Renal Physiol. 2012;302(2):F227-F233. doi:10.1152/ajprenal.00561.2011 [PMC free article: PMC3340919] [PubMed: 22031850] [CrossRef]
- 214.
- Tanner GA, Rippe C, Shao Y, Evan AP, Williams JC, Jr. Glomerular permeability to macromolecules in the Necturus kidney. Am J Physiol Renal Physiol. 2009;296(6):F1269-F1278. doi:10.1152/ajprenal.00371.2007 [PubMed: 19339627] [CrossRef]
- 215.
- Tanner GA. Glomerular sieving coefficient of serum albumin in the rat: a two-photon microscopy study. Am J Physiol Renal Physiol. 2009;296(6):F1258-F1265. doi:10.1152/ajprenal.90638.2008 [PubMed: 19211688] [CrossRef]
- 216.
- Keen H, Chlouverakis C, Fuller J, Jarrett RJ. The concomitants of raised blood sugar: studies in newly detected hyperglycemics. II. Urinary albumin excretion, blood pressure and their relation to blood sugar levels. Guys Hosp Rep. 1969;118(2):247-254. [PubMed: 5804067]
- 217.
- Nelson RG, Kunzelman CL, Pettitt DJ, Saad MF, Bennett PH, Knowler WC. Albuminuria in type 2 (non-insulin-dependent) diabetes mellitus and impaired glucose tolerance in Pima Indians. Diabetologia. 1989;32(12):870-876. doi:10.1007/BF00297452 [PubMed: 2612758] [CrossRef]
- 218.
- Collins VR, Dowse GK, Finch CF, Zimmet PZ, Linnane AW. Prevalence and risk factors for micro- and macroalbuminuria in diabetic subjects and entire population of Nauru. Diabetes. 1989;38(12):1602-1610. doi:10.2337/diab.38.12.1602 [PubMed: 2583379] [CrossRef]
- 219.
- Saydah SH, Pavkov ME, Zhang C, et al. Albuminuria prevalence in first morning void compared with previous random urine from adults in the National Health and Nutrition Examination Survey, 2009–2010. Clin Chem. 2013;59(4):675-683. doi:10.1373/clinchem.2012.195644 [PMC free article: PMC4562457] [PubMed: 23315482] [CrossRef]
- 220.
- Claudi T, Cooper JG. Comparison of urinary albumin excretion rate in overnight urine and albumin creatinine ratio in spot urine in diabetic patients in general practice. Scand J Prim Health Care. 2001;19(4):247-248. doi:10.1080/02813430152706774 [PubMed: 11822649] [CrossRef]
- 221.
- Gansevoort RT, Verhave JC, Hillege HL, et al. The validity of screening based on spot morning urine samples to detect subjects with microalbuminuria in the general population. Kidney Int Suppl. 2005;67(Suppl 94):S28-S35. doi:10.1111/j.1523-1755.2005.09408.x [PubMed: 15752236] [CrossRef]
- 222.
- Howey JE, Browning MC, Fraser CG. Biologic variation of urinary albumin: consequences for analysis, specimen collection, interpretation of results, and screening programs. Am J Kidney Dis. 1989;13(1):35-37. doi:10.1016/s0272-6386(89)80112-x [PubMed: 2912063] [CrossRef]
- 223.
- Afkarian M, Zelnick LR, Hall YN, et al. Clinical manifestations of kidney disease among US adults with diabetes, 1988–2014. JAMA. 2016;316(6):602-610. doi:10.1001/jama.2016.10924 [PMC free article: PMC5444809] [PubMed: 27532915] [CrossRef]
- 224.
- Menke A, Orchard TJ, Imperatore G, Bullard KM, Mayer-Davis E, Cowie CC. The prevalence of type 1 diabetes in the United States. Epidemiology. 2013;24(5):773-774. doi:10.1097/EDE.0b013e31829ef01a [PMC free article: PMC4562437] [PubMed: 23903880] [CrossRef]
- 225.
- Parving HH, Lewis JB, Ravid M, Remuzzi G, Hunsicker LG, DEMAND Investigators. Prevalence and risk factors for microalbuminuria in a referred cohort of type II diabetic patients: a global perspective. Kidney Int. 2006;69(11):2057-2063. doi:10.1038/sj.ki.5000377 [PubMed: 16612330] [CrossRef]
- 226.
- Shikata K, Kodera R, Utsunomiya K, et al. Prevalence of albuminuria and renal dysfunction, and related clinical factors in Japanese patients with diabetes: the Japan Diabetes Complication and its Prevention prospective study 5. J Diabetes Investig. 2020;11(2):325-332. doi:10.1111/jdi.13116 [PMC free article: PMC7078093] [PubMed: 31317670] [CrossRef]
- 227.
- Lee ES, Tang WE. The prevalence of albuminuria among diabetic patients in a primary care setting in Singapore. Singapore Med J. 2015;56(12):681-686. doi:10.11622/smedj.2015189 [PMC free article: PMC4678408] [PubMed: 26702164] [CrossRef]
- 228.
- Mogensen CE, Christensen CK. Predicting diabetic nephropathy in insulin-dependent patients. N Engl J Med. 1984;311(2):89-93. doi:10.1056/NEJM198407123110204 [PubMed: 6738599] [CrossRef]
- 229.
- Viberti GC, Jarrett RJ, Mahmud U, Hill RD, Argyropoulos A, Keen H. Microalbuminuria as a predictor of clinical nephropathy in insulin-dependent diabetes mellitus. Lancet. 1982;1(8287):1430-1432. doi:10.1016/s0140-6736(82)92450-3 [PubMed: 6123720] [CrossRef]
- 230.
- Parving HH, Oxenbøll B, Svendsen PA, Christiansen JS, Andersen AR. Early detection of patients at risk of developing diabetic nephropathy. A longitudinal study of urinary albumin excretion. Acta Endocrinol (Copenh). 1982;100(4):550-555. doi:10.1530/acta.0.1000550 [PubMed: 6812342] [CrossRef]
- 231.
- Mathiesen ER, Oxenbøll B, Johansen K, Svendsen PA, Deckert T. Incipient nephropathy in type 1 (insulin-dependent) diabetes. Diabetologia. 1984;26(6):406-410. doi:10.1007/BF00262210 [PubMed: 6468791] [CrossRef]
- 232.
- Messent JW, Elliott TG, Hill RD, Jarrett RJ, Keen H, Viberti GC. Prognostic significance of microalbuminuria in insulin-dependent diabetes mellitus: a twenty-three year follow-up study. Kidney Int. 1992;41(4):836-839. doi:10.1038/ki.1992.128 [PubMed: 1513106] [CrossRef]
- 233.
- Mogensen CE. Microalbuminuria predicts clinical proteinuria and early mortality in maturity-onset diabetes. N Engl J Med. 1984;310(6):356-360. doi:10.1056/NEJM198402093100605 [PubMed: 6690964] [CrossRef]
- 234.
- Jerums G, Cooper ME, Seeman E, Murray RM, McNeil JJ. Spectrum of proteinuria in type I and type II diabetes. Diabetes Care. 1987;10(4):419-427. doi:10.2337/diacare.10.4.419 [PubMed: 3622199] [CrossRef]
- 235.
- Nelson RG, Knowler WC, Pettitt DJ, Saad MF, Charles MA, Bennett PH. Assessment of risk of overt nephropathy in diabetic patients from albumin excretion in untimed urine specimens. Arch Intern Med. 1991;151(9):1761-1765. doi:10.1001/archinte.1991.00400090057011 [PubMed: 1888242] [CrossRef]
- 236.
- John L, Sunder PSS, Kanagasabapathy AS. Rate of progression of albuminuria in type II diabetes: five-year prospective study from south India. Diabetes Care. 1994;17(8):888-890. doi:10.2337/diacare.17.8.888 [PubMed: 7956637] [CrossRef]
- 237.
- Andersen AR, Christiansen JS, Andersen JK, Kreiner S, Deckert T. Diabetic nephropathy in type 1 (insulin-dependent) diabetes: an epidemiological study. Diabetologia. 1983;25(6):496-501. doi:10.1007/BF00284458 [PubMed: 6363177] [CrossRef]
- 238.
- Klein R, Klein BE, Moss SE. The incidence of gross proteinuria in people with insulin-dependent diabetes mellitus. Arch Intern Med. 1991;151(7):1344-1348. doi:10.1001/archinte.1991.00400070108013 [PubMed: 2064485] [CrossRef]
- 239.
- Noth RH, Krolewski AS, Kaysen GA, Meyer TW, Schambelan M. Diabetic nephropathy: hemodynamic basis and implications for disease management. Ann Intern Med. 1989;110(10):795-813. doi:10.7326/0003-4819-110-10-795 [PubMed: 2653154] [CrossRef]
- 240.
- Skrivarhaug T, Bangstad HJ, Stene LC, Sandvik L, Hanssen KF, Joner G. Low risk of overt nephropathy after 24 yr of childhood-onset type 1 diabetes mellitus (T1DM) in Norway. Pediatr Diabetes. 2006;7(5):239-246. doi:10.1111/j.1399-5448.2006.00204.x [PubMed: 17054444] [CrossRef]
- 241.
- Rossing P, Hougaard P, Parving HH. Progression of microalbuminuria in type 1 diabetes: ten-year prospective observational study. Kidney Int. 2005;68(4):1446-1450. doi:10.1111/j.1523-1755.2005.00556.x [PubMed: 16164620] [CrossRef]
- 242.
- Ballard DJ, Humphrey LL, Melton LJ, 3rd, et al. Epidemiology of persistent proteinuria in type II diabetes mellitus. Population-based study in Rochester, Minnesota. Diabetes. 1988;37(4):405-412. doi:10.2337/diab.37.4.405 [PubMed: 3378684] [CrossRef]
- 243.
- Klein R, Klein BE, Moss SE. Incidence of gross proteinuria in older-onset diabetes. A population-based perspective. Diabetes. 1993;42(3):381-389. doi:10.2337/diab.42.3.381 [PubMed: 8432408] [CrossRef]
- 244.
- Kunzelman CL, Knowler WC, Pettitt DJ, Bennett PH. Incidence of proteinuria in type 2 diabetes mellitus in the Pima Indians. Kidney Int. 1989;35(2):681-687. doi:10.1038/ki.1989.39 [PubMed: 2785225] [CrossRef]
- 245.
- Krolewski AS, Warram JH, Christlieb AR, Busick EJ, Kahn CR. The changing natural history of nephropathy in type I diabetes. Am J Med. 1985;78(5):785-794. doi:10.1016/0002-9343(85)90284-0 [PubMed: 3993659] [CrossRef]
- 246.
- Kofoed-Enevoldsen A, Borch-Johnsen K, Kreiner S, Nerup J, Deckert T. Declining incidence of persistent proteinuria in type I (insulin-dependent) diabetic patients in Denmark. Diabetes. 1987;36(2):205-209. doi:10.2337/diab.36.2.205 [PubMed: 3803732] [CrossRef]
- 247.
- Pambianco G, Costacou T, Ellis D, Becker DJ, Klein R, Orchard TJ. The 30-year natural history of type 1 diabetes complications: the Pittsburgh Epidemiology of Diabetes Complications Study experience. Diabetes. 2006;55(5):1463-1469. doi:10.2337/db05-1423 [PubMed: 16644706] [CrossRef]
- 248.
- Bojestig M, Arnqvist HJ, Hermansson G, Karlberg BE, Ludvigsson J. Declining incidence of nephropathy in insulin-dependent diabetes mellitus. N Engl J Med. 1994;330(1):15-18. doi:10.1056/NEJM199401063300103 [PubMed: 8259139] [CrossRef]
- 249.
- Rossing P. The changing epidemiology of diabetic microangiopathy in type 1 diabetes. Diabetologia. 2005;48(8):1439-1444. doi:10.1007/s00125-005-1836-x [PubMed: 15986235] [CrossRef]
- 250.
- Hovind P, Tarnow L, Rossing K, et al. Decreasing incidence of severe diabetic microangiopathy in type 1 diabetes. Diabetes Care. 2003;26(4):1258-1264. doi:10.2337/diacare.26.4.1258 [PubMed: 12663607] [CrossRef]
- 251.
- Thomas MC, Brownlee M, Susztak K, et al. Diabetic kidney disease. Nat Rev Dis Primers. 2015;1:15018. doi:10.1038/nrdp.2015.18 [PMC free article: PMC7724636] [PubMed: 27188921] [CrossRef]
- 252.
- Diabetes Control and Complications Trial Research Group, Nathan DM, Genuth S, et al. The effect of intensive treatment of diabetes on the development and progression of long-term complications in insulin-dependent diabetes mellitus. N Engl J Med. 1993;329(14):977-986. doi:10.1056/NEJM199309303291401 [PubMed: 8366922] [CrossRef]
- 253.
- Larson TS, Santanello N, Shahinfar S, et al. Trends in persistent proteinuria in adult-onset diabetes: a population-based study. Diabetes Care. 2000;23(1):51-56. doi:10.2337/diacare.23.1.51 [PubMed: 10857968] [CrossRef]
- 254.
- Pavkov ME, Knowler WC, Bennett PH, Looker HC, Krakoff J, Nelson RG. Increasing incidence of proteinuria and declining incidence of end-stage renal disease in diabetic Pima Indians. Kidney Int. 2006;70(10):1840-1846. doi:10.1038/sj.ki.5001882 [PubMed: 17003816] [CrossRef]
- 255.
- Adler AI, Stevens RJ, Manley SE, et al. Development and progression of nephropathy in type 2 diabetes: the United Kingdom Prospective Diabetes Study (UKPDS 64). Kidney Int. 2003;63(1):225-232. doi:10.1046/j.1523-1755.2003.00712.x [PubMed: 12472787] [CrossRef]
- 256.
- Bilous R. Microvascular disease: what does the UKPDS tell us about diabetic nephropathy? Diabet Med. 2008;25(Suppl 2):25-29. doi:10.1111/j.1464-5491.2008.02496.x [PubMed: 18717975] [CrossRef]
- 257.
- Kussman MJ, Goldstein H, Gleason RE. The clinical course of diabetic nephropathy. JAMA. 1976;236(16):1861-1863. doi:10.1001/jama.1976.03270170027020 [PubMed: 989537] [CrossRef]
- 258.
- Rossing P, Hommel E, Smidt UM, Parving HH. Impact of arterial blood pressure and albuminuria on the progression of diabetic nephropathy in IDDM patients. Diabetes. 1993;42(5):715-719. doi:10.2337/diab.42.5.715 [PubMed: 8482428] [CrossRef]
- 259.
- Roy MS, Affouf M, Roy A. Six-year incidence of proteinuria in type 1 diabetic African Americans. Diabetes Care. 2007;30(7):1807-1812. doi:10.2337/dc06-2534 [PubMed: 17475942] [CrossRef]
- 260.
- Roy MS, Affouf M. Six-year progression of retinopathy and associated risk factors in African American patients with type 1 diabetes mellitus: the New Jersey 725. Arch Ophthalmol. 2006;124(9):1297-1306. doi:10.1001/archopht.124.9.1297 [PubMed: 16966625] [CrossRef]
- 261.
- Araki S, Haneda M, Sugimoto T, et al. Factors associated with frequent remission of microalbuminuria in patients with type 2 diabetes. Diabetes. 2005;54(10):2983-2987. doi:10.2337/diabetes.54.10.2983 [PubMed: 16186402] [CrossRef]
- 262.
- Gaede P, Tarnow L, Vedel P, Parving HH, Pedersen O. Remission to normoalbuminuria during multifactorial treatment preserves kidney function in patients with type 2 diabetes and microalbuminuria. Nephrol Dial Transplant. 2004;19(11):2784-2788. doi:10.1093/ndt/gfh470 [PubMed: 15328385] [CrossRef]
- 263.
- Pavkov ME, Knowler WC, Hanson RL, Bennett PH, Nelson RG. Predictive power of sequential measures of albuminuria for progression to ESRD or death in Pima Indians with type 2 diabetes. Am J Kidney Dis. 2008;51(5):759-766. doi:10.1053/j.ajkd.2008.01.011 [PMC free article: PMC2386511] [PubMed: 18436086] [CrossRef]
- 264.
- Xu J, Lee ET, Devereux RB, et al. A longitudinal study of risk factors for incident albuminuria in diabetic American Indians: the Strong Heart Study. Am J Kidney Dis. 2008;51(3):415-424. doi:10.1053/j.ajkd.2007.11.010 [PMC free article: PMC2776644] [PubMed: 18295057] [CrossRef]
- 265.
- Chen C, Wang C, Hu C, et al. Normoalbuminuric diabetic kidney disease. Front Med. 2017;11(3):310-318. doi:10.1007/s11684-017-0542-7 [PubMed: 28721497] [CrossRef]
- 266.
- Kahkoska AR, Isom S, Divers J, et al. The early natural history of albuminuria in young adults with youth-onset type 1 and type 2 diabetes. J Diabetes Complications. 2018;32(12):1160-1168. doi:10.1016/j.jdiacomp.2018.09.018 [PMC free article: PMC6289668] [PubMed: 30316542] [CrossRef]
- 267.
- Xu J, Knowler WC, Devereux RB, et al. Albuminuria within the “normal” range and risk of cardiovascular disease and death in American Indians: the Strong Heart Study. Am J Kidney Dis. 2007;49(2):208-216. doi:10.1053/j.ajkd.2006.10.017 [PubMed: 17261423] [CrossRef]
- 268.
- Gerstein HC, Mann JF, Yi Q, et al. Albuminuria and risk of cardiovascular events, death, and heart failure in diabetic and nondiabetic individuals. JAMA. 2001;286(4):421-426. doi:10.1001/jama.286.4.421 [PubMed: 11466120] [CrossRef]
- 269.
- Yuyun MF, Adler AI, Wareham NJ. What is the evidence that microalbuminuria is a predictor of cardiovascular disease events? Curr Opin Nephrol Hypertens. 2005;14(3):271-276. doi:10.1097/01.mnh.0000165895.90748.3b [PubMed: 15821422] [CrossRef]
- 270.
- Hillege HL, Fidler V, Diercks GF, et al. Urinary albumin excretion predicts cardiovascular and noncardiovascular mortality in general population. Circulation. 2002;106(14):1777-1782. doi:10.1161/01.cir.0000031732.78052.81 [PubMed: 12356629] [CrossRef]
- 271.
- Astor BC, Hallan SI, Miller ER, 3rd, Yeung E, Coresh J. Glomerular filtration rate, albuminuria, and risk of cardiovascular and all-cause mortality in the US population. Am J Epidemiol. 2008;167(10):1226-1234. doi:10.1093/aje/kwn033 [PubMed: 18385206] [CrossRef]
- 272.
- Borch-Johnsen K, Andersen PK, Deckert T. The effect of proteinuria on relative mortality in type 1 (insulin-dependent) diabetes mellitus. Diabetologia. 1985;28(8):590-596. doi:10.1007/BF00281993 [PubMed: 4054448] [CrossRef]
- 273.
- Nelson RG, Pettitt DJ, Carraher MJ, Baird HR, Knowler WC. Effect of proteinuria on mortality in NIDDM. Diabetes. 1988;37(11):1499-1504. doi:10.2337/diab.37.11.1499 [PubMed: 3181642] [CrossRef]
- 274.
- Jensen T, Borch-Johnsen K, Kofoed-Enevoldsen A, Deckert T. Coronary heart disease in young type 1 (insulin-dependent) diabetic patients with and without diabetic nephropathy: incidence and risk factors. Diabetologia. 1987;30(3):144-148. doi:10.1007/BF00274218 [PubMed: 3582820] [CrossRef]
- 275.
- Jarrett RJ. The epidemiology of coronary heart disease and related factors in the context of diabetes mellitus and impaired glucose tolerance. In: Jarrett RJ, ed. Diabetes and Heart Disease. Elsevier; 1984:1-23.
- 276.
- Pavkov ME, Bennett PH, Sievers ML, et al. Predominant effect of kidney disease on mortality in Pima Indians with or without type 2 diabetes. Kidney Int. 2005;68(3):1267-1274. doi:10.1111/j.1523-1755.2005.00523.x [PMC free article: PMC1800940] [PubMed: 16105060] [CrossRef]
- 277.
- Orchard TJ, Secrest AM, Miller RG, Costacou T. In the absence of renal disease, 20 year mortality risk in type 1 diabetes is comparable to that of the general population: a report from the Pittsburgh Epidemiology of Diabetes Complications Study. Diabetologia. 2010;53(11):2312-2319. doi:10.1007/s00125-010-1860-3 [PMC free article: PMC3057031] [PubMed: 20665208] [CrossRef]
- 278.
- Arun CS, Stoddart J, Mackin P, MacLeod JM, New JP, Marshall SM. Significance of microalbuminuria in long-duration type 1 diabetes. Diabetes Care. 2003;26(7):2144-2149. doi:10.2337/diacare.26.7.2144 [PubMed: 12832327] [CrossRef]
- 279.
- Allen KV, Walker JD. Microalbuminuria and mortality in long-duration type 1 diabetes. Diabetes Care. 2003;26(8):2389-2391. doi:10.2337/diacare.26.8.2389 [PubMed: 12882867] [CrossRef]
- 280.
- Rosolowsky ET, Skupien J, Smiles AM, et al. Risk for ESRD in type 1 diabetes remains high despite renoprotection. J Am Soc Nephrol. 2011;22(3):545-553. doi:10.1681/ASN.2010040354 [PMC free article: PMC3060448] [PubMed: 21355053] [CrossRef]
- 281.
- Nelson RG. Is treatment of nephropathy in type 1 diabetes efficacious but ineffective? J Am Soc Nephrol. 2011;22(3):402-404. doi:10.1681/ASN.2011010076 [PubMed: 21355059] [CrossRef]
- 282.
- Groop PH, Thomas MC, Moran JL, et al. The presence and severity of chronic kidney disease predicts all-cause mortality in type 1 diabetes. Diabetes. 2009;58(7):1651-1658. doi:10.2337/db08-1543 [PMC free article: PMC2699848] [PubMed: 19401416] [CrossRef]
- 283.
- Perrone RD, Madias NE, Levey AS. Serum creatinine as an index of renal function: new insights into old concepts. Clin Chem. 1992;38(10):1933-1953. doi:10.1093/clinchem/38.10.1933 [PubMed: 1394976] [CrossRef]
- 284.
- Jarrett RJ, Viberti GC, Argyropoulos A, Hill RD, Mahmud U, Murrells TJ. Microalbuminuria predicts mortality in non-insulin-dependent diabetes. Diabet Med. 1984;1(1):17-19. doi:10.1111/j.1464-5491.1984.tb01915.x [PubMed: 6242771] [CrossRef]
- 285.
- Schmitz A, Vaeth M. Microalbuminuria: a major risk factor in non-insulin-dependent diabetes. A 10-year follow-up study of 503 patients. Diabet Med. 1988;5(2):126-134. doi:10.1111/j.1464-5491.1988.tb00958.x [PubMed: 2964977] [CrossRef]
- 286.
- Mattock MB, Morrish NJ, Viberti GC, Keen H, Fitzgerald AP, Jackson G. Prospective study of microalbuminuria as predictor of mortality in NIDDM. Diabetes. 1992;41(6):736-741. doi:10.2337/diab.41.6.736 [PubMed: 1587400] [CrossRef]
- 287.
- Neil A, Hawkins M, Potok M, Thorogood M, Cohen D, Mann J. A prospective population-based study of microalbuminuria as a predictor of mortality in NIDDM. Diabetes Care. 1993;16(7):996-1003. doi:10.2337/diacare.16.7.996 [PubMed: 8359108] [CrossRef]
- 288.
- Shara NM, Wang H, Mete M, et al. Estimated GFR and incident cardiovascular disease events in American Indians: the Strong Heart Study. Am J Kidney Dis. 2012;60(5):795-803. doi:10.1053/j.ajkd.2012.06.015 [PMC free article: PMC3473098] [PubMed: 22841159] [CrossRef]
- 289.
- Naqvi SB, Collins AJ. Infectious complications in chronic kidney disease. Adv Chronic Kidney Dis. 2006;13(3):199-204. doi:10.1053/j.ackd.2006.04.004 [PubMed: 16815225] [CrossRef]
- 290.
- McDonald HI, Thomas SL, Millett ER, Nitsch D. CKD and the risk of acute, community-acquired infections among older people with diabetes mellitus: a retrospective cohort study using electronic health records. Am J Kidney Dis. 2015;66(1):60-68. doi:10.1053/j.ajkd.2014.11.027 [PMC free article: PMC4510204] [PubMed: 25641062] [CrossRef]
- 291.
- Dalrymple LS, Katz R, Kestenbaum B, et al. The risk of infection-related hospitalization with decreased kidney function. Am J Kidney Dis. 2012;59(3):356-363. doi:10.1053/j.ajkd.2011.07.012 [PMC free article: PMC3288732] [PubMed: 21906862] [CrossRef]
- 292.
- Shebl FM, Warren JL, Eggers PW, Engels EA. Cancer risk among elderly persons with end-stage renal disease: a population-based case-control study. BMC Nephrol. 2012;13:65. doi:10.1186/1471-2369-13-65 [PMC free article: PMC3441292] [PubMed: 22834953] [CrossRef]
- 293.
- Wong G, Hayen A, Chapman JR, et al. Association of CKD and cancer risk in older people. J Am Soc Nephrol. 2009;20(6):1341-1350. doi:10.1681/ASN.2008090998 [PMC free article: PMC2689896] [PubMed: 19406977] [CrossRef]
- 294.
- Kitchlu A, Reid J, Jeyakumar N, et al. Cancer risk and mortality in patients with kidney disease: a population-based cohort study. Am J Kidney Dis. 2022;80(4):436-448.e1. doi:10.1053/j.ajkd.2022.02.020 [PubMed: 35405208] [CrossRef]
- 295.
- Larsson SC, Wolk A. Diabetes mellitus and incidence of kidney cancer: a meta-analysis of cohort studies. Diabetologia. 2011;54(5):1013-1018. doi:10.1007/s00125-011-2051-6 [PubMed: 21274512] [CrossRef]
- 296.
- Habib SL, Rojna M. Diabetes and risk of cancer. ISRN Oncol. 2013;2013:583786. doi:10.1155/2013/583786 [PMC free article: PMC3582053] [PubMed: 23476808] [CrossRef]
- 297.
- Izzedine H, Perazella MA. Onco-nephrology: an appraisal of the cancer and chronic kidney disease links. Nephrol Dial Transplant. 2015;30(12):1979-1988. doi:10.1093/ndt/gfu387 [PMC free article: PMC4832985] [PubMed: 25648910] [CrossRef]
- 298.
- Berhane AM, Weil EJ, Knowler WC, Nelson RG, Hanson RL. Albuminuria and estimated glomerular filtration rate as predictors of diabetic end-stage renal disease and death. Clin J Am Soc Nephrol. 2011;6(10):2444-2451. doi:10.2215/CJN.00580111 [PMC free article: PMC3186453] [PubMed: 21852671] [CrossRef]
- 299.
- Ninomiya T, Perkovic V, de Galan BE, et al. Albuminuria and kidney function independently predict cardiovascular and renal outcomes in diabetes. J Am Soc Nephrol. 2009;20(8):1813-1821. doi:10.1681/asn.2008121270 [PMC free article: PMC2723977] [PubMed: 19443635] [CrossRef]
- 300.
- Shara NM, Wang H, Valaitis E, et al. Comparison of estimated glomerular filtration rates and albuminuria in predicting risk of coronary heart disease in a population with high prevalence of diabetes mellitus and renal disease. Am J Cardiol. 2011;107(3):399-405. doi:10.1016/j.amjcard.2010.09.036 [PMC free article: PMC3035999] [PubMed: 21257005] [CrossRef]
- 301.
- Rosenson RS, Fioretto P, Dodson PM. Does microvascular disease predict macrovascular events in type 2 diabetes? Atherosclerosis. 2011;218(1):13-18. doi:10.1016/j.atherosclerosis.2011.06.029 [PubMed: 21763654] [CrossRef]
- 302.
- Yokoyama H, Oishi M, Kawai K, Sone H, Japan Diabetes Clinical Data Management Study Group. Reduced GFR and microalbuminuria are independently associated with prevalent cardiovascular disease in type 2 diabetes: JDDM study 16. Diabet Med. 2008;25(12):1426-1432. doi:10.1111/j.1464-5491.2008.02592.x [PubMed: 19046241] [CrossRef]
- 303.
- Tong PC, Kong AP, So WY, et al. Interactive effect of retinopathy and macroalbuminuria on all-cause mortality, cardiovascular and renal end points in Chinese patients with type 2 diabetes mellitus. Diabet Med. 2007;24(7):741-746. doi:10.1111/j.1464-5491.2007.02145.x [PubMed: 17403120] [CrossRef]
- 304.
- So WY, Kong AP, Ma RC, et al. Glomerular filtration rate, cardiorenal end points, and all-cause mortality in type 2 diabetic patients. Diabetes Care. 2006;29(9):2046-2052. doi:10.2337/dc06-0248 [PubMed: 16936151] [CrossRef]
- 305.
- Gimeno-Orna JA, Lou-Arnal LM, Boned-Juliani B, Molinero-Herguedas E. Mild renal insufficiency as a cardiovascular risk factor in non-proteinuric type II diabetes. Diabetes Res Clin Pract. 2004;64(3):191-199. doi:10.1016/j.diabres.2003.10.018 [PubMed: 15126007] [CrossRef]
- 306.
- Yang X, Ma RC, So WY, et al. Impacts of chronic kidney disease and albuminuria on associations between coronary heart disease and its traditional risk factors in type 2 diabetic patients—the Hong Kong Diabetes Registry. Cardiovasc Diabetol. 2007;6:37. doi:10.1186/1475-2840-6-37 [PMC free article: PMC2219954] [PubMed: 18053157] [CrossRef]
- 307.
- Leelawattana R, Rattarasarn C, Lim A, Soonthornpun S, Setasuban W. Causes of death, incidence and risk factors of cardiovascular diseases in Thai type 2 diabetic patients: a 5 year follow-up study. Diabetes Res Clin Pract. 2003;60(3):183-189. doi:10.1016/s0168-8227(03)00035-4 [PubMed: 12822563] [CrossRef]
- 308.
- Juutilainen A, Lehto S, Rönnemaa T, Pyörälä K, Laakso M. Proteinuria and metabolic syndrome as predictors of cardiovascular death in non-diabetic and type 2 diabetic men and women. Diabetologia. 2006;49(1):56-65. doi:10.1007/s00125-005-0050-1 [PubMed: 16365726] [CrossRef]
- 309.
- Bruno G, Merletti F, Bargero G, et al. Estimated glomerular filtration rate, albuminuria and mortality in type 2 diabetes: the Casale Monferrato study. Diabetologia. 2007;50(5):941-948. doi:10.1007/s00125-007-0616-1 [PubMed: 17333106] [CrossRef]
- 310.
- Casiglia E, Zanette G, Mazza A, et al. Cardiovascular mortality in non-insulin-dependent diabetes mellitus. A controlled study among 683 diabetics and 683 age- and sex-matched normal subjects. Eur J Epidemiol. 2000;16(7):677-684. doi:10.1023/A:1007673123716 [PubMed: 11078126] [CrossRef]
- 311.
- Valmadrid CT, Klein R, Moss SE, Klein BE. The risk of cardiovascular disease mortality associated with microalbuminuria and gross proteinuria in persons with older-onset diabetes mellitus. Arch Intern Med. 2000;160(8):1093-1100. doi:10.1001/archinte.160.8.1093 [PubMed: 10789601] [CrossRef]
- 312.
- Yang X, So WY, Kong AP, et al. Development and validation of stroke risk equation for Hong Kong Chinese patients with type 2 diabetes: the Hong Kong Diabetes Registry. Diabetes Care. 2007;30(1):65-70. doi:10.2337/dc06-1273 [PubMed: 17192335] [CrossRef]
- 313.
- Davis TM, Millns H, Stratton IM, Holman RR, Turner RC. Risk factors for stroke in type 2 diabetes mellitus: United Kingdom Prospective Diabetes Study (UKPDS) 29. Arch Intern Med. 1999;159(10):1097-1103. doi:10.1001/archinte.159.10.1097 [PubMed: 10335687] [CrossRef]
- 314.
- Knobler H, Zornitzki T, Vered S, et al. Reduced glomerular filtration rate in asymptomatic diabetic patients: predictor of increased risk for cardiac events independent of albuminuria. J Am Coll Cardiol. 2004;44(11):2142-2148. doi:10.1016/j.jacc.2004.09.006 [PubMed: 15582311] [CrossRef]
- 315.
- Fox CS, Matsushita K, Woodward M, et al. Associations of kidney disease measures with mortality and end-stage renal disease in individuals with and without diabetes: a meta-analysis. Lancet. 2012;380(9854):1662-1673. doi:10.1016/S0140-6736(12)61350-6 [PMC free article: PMC3771350] [PubMed: 23013602] [CrossRef]
- 316.
- Muntner P, Coresh J, Powe NR, Klag MJ. The contribution of increased diabetes prevalence and improved myocardial infarction and stroke survival to the increase in treated end-stage renal disease. J Am Soc Nephrol. 2003;14(6):1568-1577. doi:10.1097/01.asn.0000067420.83632.c1 [PubMed: 12761257] [CrossRef]
- 317.
- Burrows NR, Zhang Y, Hora I, et al. Sustained lower incidence of diabetes-related end-stage kidney disease among American Indians and Alaska Natives, Blacks, and Hispanics in the U.S., 2000–2016. Diabetes Care. 2020;43(9):2090-2097. doi:10.2337/dc20-0495 [PMC free article: PMC8628545] [PubMed: 32616609] [CrossRef]
- 318.
- Chu CD, Powe NR, McCulloch CE, et al. Trends in chronic kidney disease care in the US by race and ethnicity, 2012–2019. JAMA Netw Open. 2021;4(9):e2127014. doi:10.1001/jamanetworkopen.2021.27014 [PMC free article: PMC8477264] [PubMed: 34570204] [CrossRef]
- 319.
- Cowie CC, Port FK, Wolfe RA, Savage PJ, Moll PP, Hawthorne VM. Disparities in incidence of diabetic end-stage renal disease according to race and type of diabetes. N Engl J Med. 1989;321(16):1074-1079. doi:10.1056/NEJM198910193211603 [PubMed: 2797067] [CrossRef]
- 320.
- Nelson RG, Newman JM, Knowler WC, et al. Incidence of end-stage renal disease in type 2 (non-insulin-dependent) diabetes mellitus in Pima Indians. Diabetologia. 1988;31(10):730-736. doi:10.1007/BF00274774 [PubMed: 3240833] [CrossRef]
- 321.
- Rostand SG, Kirk KA, Rutsky EA, Pate BA. Racial differences in the incidence of treatment for end-stage renal disease. N Engl J Med. 1982;306(21):1276-1279. doi:10.1056/NEJM198205273062106 [PubMed: 7040967] [CrossRef]
- 322.
- Lopes AA, Port FK, James SA, Agodoa L. The excess risk of treated end-stage renal disease in Blacks in the United States. J Am Soc Nephrol. 1993;3(12):1961-1971. doi:10.1681/ASN.V3121961 [PubMed: 8338929] [CrossRef]
- 323.
- Stephens GW, Gillaspy JA, Clyne D, Mejia A, Pollak VE. Racial differences in the incidence of end-stage renal disease in types I and II diabetes mellitus. Am J Kidney Dis. 1990;15(6):562-567. doi:10.1016/s0272-6386(12)80527-0 [PubMed: 2368696] [CrossRef]
- 324.
- Pugh JA, Stern MP, Haffner SM, Eifler CW, Zapata M. Excess incidence of treatment of end-stage renal disease in Mexican Americans. Am J Epidemiol. 1988;127(1):135-144. doi:10.1093/oxfordjournals.aje.a114773 [PubMed: 3276155] [CrossRef]
- 325.
- Burden AC, McNally PG, Feehally J, Walls J. Increased incidence of end-stage renal failure secondary to diabetes mellitus in Asian ethnic groups in the United Kingdom. Diabet Med. 1992;9(7):641-645. doi:10.1111/j.1464-5491.1992.tb01860.x [PubMed: 1511571] [CrossRef]
- 326.
- Newman JM, Marfin AA, Eggers PW, Helgerson SD. End state renal disease among Native Americans, 1983–86. Am J Public Health. 1990;80(3):318-319. doi:10.2105/ajph.80.3.318 [PMC free article: PMC1404694] [PubMed: 2305914] [CrossRef]
- 327.
- Pavkov ME, Bennett PH, Knowler WC, Krakoff J, Sievers ML, Nelson RG. Effect of youth-onset type 2 diabetes mellitus on incidence of end-stage renal disease and mortality in young and middle-aged Pima Indians. JAMA. 2006;296(4):421-426. doi:10.1001/jama.296.4.421 [PubMed: 16868300] [CrossRef]
- 328.
- Pavkov ME, Hanson RL, Knowler WC, Sievers ML, Bennett PH, Nelson RG. Effect of intrauterine diabetes exposure on the incidence of end-stage renal disease in young adults with type 2 diabetes. Diabetes Care. 2010;33(11):2396-2398. doi:10.2337/dc10-0811 [PMC free article: PMC2963501] [PubMed: 20693350] [CrossRef]
- 329.
- McMillan MA, Briggs JD, Junor BJ. Outcome of renal replacement treatment in patients with diabetes mellitus. BMJ. 1990;301(6751):540-544. doi:10.1136/bmj.301.6751.540 [PMC free article: PMC1663846] [PubMed: 2207427] [CrossRef]
- 330.
- Hirschl MM, Heinz G, Sunder-Plassmann G, Derfler K. Renal replacement therapy in type 2 diabetic patients: 10 years’ experience. Am J Kidney Dis. 1992;20(6):564-568. doi:10.1016/s0272-6386(12)70219-6 [PubMed: 1462983] [CrossRef]
- 331.
- Rischen-Vos J, van der Woude FJ, Tegzess AM, et al. Increased morbidity and mortality in patients with diabetes mellitus after kidney transplantation as compared with non-diabetic patients. Nephrol Dial Transplant. 1992;7(5):433-437. doi:10.1093/oxfordjournals.ndt.a092162 [PubMed: 1321380] [CrossRef]
- 332.
- Koch M, Thomas B, Tschöpe W, Ritz E. Survival and predictors of death in dialysed diabetic patients. Diabetologia. 1993;36(10):1113-1117. doi:10.1007/BF02374508 [PubMed: 8243864] [CrossRef]
- 333.
- Cowie CC, Port FK, Rust KF, Harris MI. Differences in survival between Black and White patients with diabetic end-stage renal disease. Diabetes Care. 1994;17(7):681-687. doi:10.2337/diacare.17.7.681 [PubMed: 7924777] [CrossRef]
- 334.
- Yan G, Norris KC, Yu AJ, et al. The relationship of age, race, and ethnicity with survival in dialysis patients. Clin J Am Soc Nephrol. 2013;8(6):953-961. doi:10.2215/CJN.09180912 [PMC free article: PMC3675850] [PubMed: 23539227] [CrossRef]
- 335.
- Murthy BV, Molony DA, Stack AG. Survival advantage of Hispanic patients initiating dialysis in the United States is modified by race. J Am Soc Nephrol. 2005;16(3):782-790. doi:10.1681/ASN.2004080627 [PubMed: 15677309] [CrossRef]
- 336.
- Nelson RG, Hanson RL, Pettitt DJ, Knowler WC, Bennett PH. Survival during renal replacement therapy for diabetic end-stage renal disease in Pima Indians. Diabetes Care. 1996;19(12):1333-1337. doi:10.2337/diacare.19.12.1333 [PubMed: 8941459] [CrossRef]
- 337.
- Burrows NR, Cho P, McKeever Bullard K, Narva AS, Eggers PW. Survival on dialysis among American Indians and Alaska Natives with diabetes in the United States, 1995–2010. Am J Public Health. 2014;104(Suppl 3):S490-S495. doi:10.2105/AJPH.2014.301942 [PMC free article: PMC4035878] [PubMed: 24754656] [CrossRef]
- 338.
- Humphrey LL, Ballard DJ, Frohnert PP, Chu CP, O’Fallon WM, Palumbo PJ. Chronic renal failure in non-insulin-dependent diabetes mellitus. A population-based study in Rochester, Minnesota. Ann Intern Med. 1989;111(10):788-796. doi:10.7326/0003-4819-111-10-788 [PubMed: 2817626] [CrossRef]
- 339.
- Hasslacher C, Ritz E, Wahl P, Michael C. Similar risks of nephropathy in patients with type I or type II diabetes mellitus. Nephrol Dial Transplant. 1989;4(10):859-863. doi:10.1093/ndt/4.10.859 [PubMed: 2515489] [CrossRef]
- 340.
- Pugh JA, Medina R, Ramirez M. Comparison of the course to end-stage renal disease of type 1 (insulin-dependent) and type 2 (non-insulin-dependent) diabetic nephropathy. Diabetologia. 1993;36(10):1094-1098. doi:10.1007/BF02374504 [PubMed: 8243860] [CrossRef]
- 341.
- Piani F, Melena I, Tommerdahl KL, et al. Sex-related differences in diabetic kidney disease: a review on the mechanisms and potential therapeutic implications. J Diabetes Complications. 2021;35(4):107841. doi:10.1016/j.jdiacomp.2020.107841 [PMC free article: PMC8007279] [PubMed: 33423908] [CrossRef]
- 342.
- Norris K, Nissenson AR. Race, gender, and socioeconomic disparities in CKD in the United States. J Am Soc Nephrol. 2008;19(7):1261-1270. doi:10.1681/ASN.2008030276 [PubMed: 18525000] [CrossRef]
- 343.
- Barbour SJ, Schachter M, Er L, Djurdjev O, Levin A. A systematic review of ethnic differences in the rate of renal progression in CKD patients. Nephrol Dial Transplant. 2010;25(8):2422-2430. doi:10.1093/ndt/gfq283 [PubMed: 20519230] [CrossRef]
- 344.
- Ward MM. Socioeconomic status and the incidence of ESRD. Am J Kidney Dis. 2008;51(4):563-572. doi:10.1053/j.ajkd.2007.11.023 [PubMed: 18371532] [CrossRef]
- 345.
- Corwin TR, Ozieh MN, Garacci E, Palatnik A, Egede LE. The relationship between financial hardship and incident diabetic kidney disease in older US adults—a longitudinal study. BMC Nephrol. 2021;22(1):167. doi:10.1186/s12882-021-02373-3 [PMC free article: PMC8101204] [PubMed: 33952186] [CrossRef]
- 346.
- Norris KC. Socioeconomic and racial disparities related to chronic kidney disease and type 2 diabetes. In: Chronic Kidney Disease and Type 2 Diabetes. eBook. American Diabetes Association; 2021. https://www
.ncbi.nlm .nih.gov/pubmed/34279874 [PubMed: 34279874] - 347.
- Hirsch AG, Nordberg CM, Chang A, et al. Association of community socioeconomic deprivation with evidence of reduced kidney function at time of type 2 diabetes diagnosis. SSM Popul Health. 2021;15:100876. doi:10.1016/j.ssmph.2021.100876 [PMC free article: PMC8327153] [PubMed: 34377762] [CrossRef]
- 348.
- Rasking L, Vanbrabant K, Bové H, et al. Adverse effects of fine particulate matter on human kidney functioning: a systematic review. Environ Health. 2022;21(1):24. doi:10.1186/s12940-021-00827-7 [PMC free article: PMC8822715] [PubMed: 35135544] [CrossRef]
- 349.
- Duru OK, Middleton T, Tewari MK, Norris K. The landscape of diabetic kidney disease in the United States. Curr Diab Rep. 2018;18(3):14. doi:10.1007/s11892-018-0980-x [PMC free article: PMC5817078] [PubMed: 29457196] [CrossRef]
- 350.
- Painter RC, Osmond C, Gluckman P, Hanson M, Phillips DI, Roseboom TJ. Transgenerational effects of prenatal exposure to the Dutch famine on neonatal adiposity and health in later life. BJOG. 2008;115(10):1243-1249. doi:10.1111/j.1471-0528.2008.01822.x [PubMed: 18715409] [CrossRef]
- 351.
- Klein R, Klein BE, Moss SE. Prevalence of microalbuminuria in older-onset diabetes. Diabetes Care. 1993;16(10):1325-1330. doi:10.2337/diacare.16.10.1325 [PubMed: 8269789] [CrossRef]
- 352.
- Coonrod BA, Ellis D, Becker DJ, et al. Predictors of microalbuminuria in individuals with IDDM. Pittsburgh Epidemiology of Diabetes Complications Study. Diabetes Care. 1993;16(10):1376-1383. doi:10.2337/diacare.16.10.1376 [PubMed: 8269796] [CrossRef]
- 353.
- Hasslacher C, Stech W, Wahl P, Ritz E. Blood pressure and metabolic control as risk factors for nephropathy in type 1 (insulin-dependent) diabetes. Diabetologia. 1985;28(1):6-11. doi:10.1007/BF00276992 [PubMed: 3979689] [CrossRef]
- 354.
- Nelson RG, Knowler WC, Pettitt DJ, Hanson RL, Bennett PH. Incidence and determinants of elevated urinary albumin excretion in Pima Indians with NIDDM. Diabetes Care. 1995;18(2):182-187. doi:10.2337/diacare.18.2.182 [PubMed: 7729295] [CrossRef]
- 355.
- Cooper ME, Frauman A, O’Brien RC, Seeman E, Murray RM, Jerums G. Progression of proteinuria in type 1 and type 2 diabetes. Diabet Med. 1988;5(4):361-368. doi:10.1111/j.1464-5491.1988.tb01006.x [PubMed: 2968887] [CrossRef]
- 356.
- Fabre J, Balant LP, Dayer PG, Fox HM, Vernet AT. The kidney in maturity onset diabetes mellitus: a clinical study of 510 patients. Kidney Int. 1982;21(5):730-738. doi:10.1038/ki.1982.90 [PubMed: 7109459] [CrossRef]
- 357.
- Niskanen L, Voutilainen R, Teräsvirta M, et al. A prospective study of clinical and metabolic associates of proteinuria in patients with type 2 diabetes mellitus. Diabet Med. 1993;10(6):543-549. doi:10.1111/j.1464-5491.1993.tb00118.x [PubMed: 8365091] [CrossRef]
- 358.
- Larkins RG, Dunlop ME. The link between hyperglycaemia and diabetic nephropathy. Diabetologia. 1992;35(6):499-504. doi:10.1007/BF00400475 [PubMed: 1612221] [CrossRef]
- 359.
- Mauer SM, Goetz FC, McHugh LE, et al. Long-term study of normal kidneys transplanted into patients with type I diabetes. Diabetes. 1989;38(4):516-523. doi:10.2337/diab.38.4.516 [PubMed: 2647558] [CrossRef]
- 360.
- Scott LJ, Warram JH, Hanna LS, Laffel LM, Ryan L, Krolewski AS. A nonlinear effect of hyperglycemia and current cigarette smoking are major determinants of the onset of microalbuminuria in type 1 diabetes. Diabetes. 2001;50(12):2842-2849. doi:10.2337/diabetes.50.12.2842 [PubMed: 11723069] [CrossRef]
- 361.
- Romero P, Salvat M, Fernández J, Baget M, Martinez I. Renal and retinal microangiopathy after 15 years of follow-up study in a sample of type 1 diabetes mellitus patients. J Diabetes Complications. 2007;21(2):93-100. doi:10.1016/j.jdiacomp.2006.04.001 [PubMed: 17331857] [CrossRef]
- 362.
- Selvin E, Francis LM, Ballantyne CM, et al. Nontraditional markers of glycemia: associations with microvascular conditions. Diabetes Care. 2011;34(4):960-967. doi:10.2337/dc10-1945 [PMC free article: PMC3064058] [PubMed: 21335368] [CrossRef]
- 363.
- Fioretto P, Bruseghin M, Berto I, Gallina P, Manzato E, Mussap M. Renal protection in diabetes: role of glycemic control. J Am Soc Nephrol. 2006;17(4 Suppl 2):S86-S89. doi:10.1681/ASN.2005121343 [PubMed: 16565255] [CrossRef]
- 364.
- Keane WF, Brenner BM, de Zeeuw D, et al. The risk of developing end-stage renal disease in patients with type 2 diabetes and nephropathy: the RENAAL study. Kidney Int. 2003;63(4):1499-1507. doi:10.1046/j.1523-1755.2003.00885.x [PubMed: 12631367] [CrossRef]
- 365.
- Wiseman MJ, Saunders AJ, Keen H, Viberti G. Effect of blood glucose control on increased glomerular filtration rate and kidney size in insulin-dependent diabetes. N Engl J Med. 1985;312(10):617-621. doi:10.1056/NEJM198503073121004 [PubMed: 3883162] [CrossRef]
- 366.
- Christiansen JS, Frandsen M, Parving HH. Effects of intravenous glucose infusion on renal function in normal man and in insulin-dependent diabetics. Diabetologia. 1981;21(4):368-373. doi:10.1007/BF00252683 [PubMed: 7286497] [CrossRef]
- 367.
- Helal I, Fick-Brosnahan GM, Reed-Gitomer B, Schrier RW. Glomerular hyperfiltration: definitions, mechanisms and clinical implications. Nat Rev Nephrol. 2012;8(5):293-300. doi:10.1038/nrneph.2012.19 [PubMed: 22349487] [CrossRef]
- 368.
- Steffes MW, Sutherland DE, Goetz FC, Rich SS, Mauer SM. Studies of kidney and muscle biopsy specimens from identical twins discordant for type I diabetes mellitus. N Engl J Med. 1985;312(20):1282-1287. doi:10.1056/NEJM198505163122003 [PubMed: 4039409] [CrossRef]
- 369.
- Fioretto P, Mauer M. Reversal of diabetic nephropathy: lessons from pancreas transplantation. J Nephrol. 2012;25(1):13-18. doi:10.5301/jn.5000061 [PubMed: 22241641] [CrossRef]
- 370.
- Mathiesen ER, Rønn B, Jensen T, Storm B, Deckert T. Relationship between blood pressure and urinary albumin excretion in development of microalbuminuria. Diabetes. 1990;39(2):245-249. doi:10.2337/diab.39.2.245 [PubMed: 2227133] [CrossRef]
- 371.
- Raal FJ, Kalk WJ, Taylor DR, Osler CE, Panz VR. The relationship between the development and progression of microalbuminuria and arterial blood pressure in type 1 (insulin-dependent) diabetes mellitus. Diabetes Res Clin Pract. 1992;16(3):221-227. doi:10.1016/0168-8227(92)90121-7 [PubMed: 1425143] [CrossRef]
- 372.
- Steinke JM. The natural progression of kidney injury in young type 1 diabetic patients. Curr Diab Rep. 2009;9(6):473-479. doi:10.1007/s11892-009-0077-7 [PubMed: 19954694] [CrossRef]
- 373.
- Schultz CJ, Neil HA, Dalton RN, Konopelska Bahu T, Dunger DB. Blood pressure does not rise before the onset of microalbuminuria in children followed from diagnosis of type 1 diabetes. Oxford Regional Prospective Study Group. Diabetes Care. 2001;24(3):555-560. doi:10.2337/diacare.24.3.555 [PubMed: 11289484] [CrossRef]
- 374.
- Viberti GC, Keen H, Wiseman MJ. Raised arterial pressure in parents of proteinuric insulin dependent diabetics. Br Med J (Clin Res Ed). 1987;295(6597):515-517. doi:10.1136/bmj.295.6597.515 [PMC free article: PMC1247425] [PubMed: 3117203] [CrossRef]
- 375.
- Barzilay J, Warram JH, Bak M, Laffel LM, Canessa M, Krolewski AS. Predisposition to hypertension: risk factor for nephropathy and hypertension in IDDM. Kidney Int. 1992;41(4):723-730. doi:10.1038/ki.1992.113 [PubMed: 1513093] [CrossRef]
- 376.
- Lurbe E, Redon J, Kesani A, et al. Increase in nocturnal blood pressure and progression to microalbuminuria in type 1 diabetes. N Engl J Med. 2002;347(11):797-805. doi:10.1056/NEJMoa013410 [PubMed: 12226150] [CrossRef]
- 377.
- Sun D, Wang J, Shao W, et al. Pathogenesis and damage targets of hypertensive kidney injury. J Transl Int Med. 2020;8(4):205-209. doi:10.2478/jtim-2020-0033 [PMC free article: PMC7805285] [PubMed: 33511047] [CrossRef]
- 378.
- Nelson RG, Pettitt DJ, de Courten MP, Hanson RL, Knowler WC, Bennett PH. Parental hypertension and proteinuria in Pima Indians with NIDDM. Diabetologia. 1996;39(4):433-438. doi:10.1007/BF00400674 [PubMed: 8777992] [CrossRef]
- 379.
- Nelson RG, Pettitt DJ, Baird HR, et al. Pre-diabetic blood pressure predicts urinary albumin excretion after the onset of type 2 (non-insulin-dependent) diabetes mellitus in Pima Indians. Diabetologia. 1993;36(10):998-1001. doi:10.1007/BF02374490 [PubMed: 8243885] [CrossRef]
- 380.
- Canessa M, Adragna N, Solomon HS, Connolly TM, Tosteson DC. Increased sodium-lithium countertransport in red cells of patients with essential hypertension. N Engl J Med. 1980;302(14):772-776. doi:10.1056/NEJM198004033021403 [PubMed: 7354809] [CrossRef]
- 381.
- Woods JW, Falk RJ, Pittman AW, Klemmer PJ, Watson BS, Namboodiri K. Increased red-cell sodium-lithium countertransport in normotensive sons of hypertensive parents. N Engl J Med. 1982;306(10):593-595. doi:10.1056/NEJM198203113061007 [PubMed: 6949036] [CrossRef]
- 382.
- Clegg G, Morgan DB, Davidson C. The heterogeneity of essential hypertension. Relation between lithium efflux and sodium content of erythrocytes and a family history of hypertension. Lancet. 1982;2(8304):891-894. doi:10.1016/s0140-6736(82)90864-9 [PubMed: 6126750] [CrossRef]
- 383.
- Cooper R, LeGrady D, Nanas S, et al. Increased sodium-lithium countertransport in college students with elevated blood pressure. JAMA. 1983;249(8):1030-1034. doi:10.1001/jama.1983.03330320028026 [PubMed: 6823057] [CrossRef]
- 384.
- Zerbini G, Gabellini D, Ruggieri D, Maestroni A. Increased sodium-lithium countertransport activity: a cellular dysfunction common to essential hypertension and diabetic nephropathy. J Am Soc Nephrol. 2004;15(Suppl 1):S81-S84. doi:10.1097/01.asn.0000093371.65105.8f [PubMed: 14684679] [CrossRef]
- 385.
- Mangili R, Bending JJ, Scott G, Li LK, Gupta A, Viberti G. Increased sodium-lithium countertransport activity in red cells of patients with insulin-dependent diabetes and nephropathy. N Engl J Med. 1988;318(3):146-150. doi:10.1056/NEJM198801213180304 [PubMed: 3336402] [CrossRef]
- 386.
- Jones SL, Trevisan R, Tariq T, et al. Sodium-lithium countertransport in microalbuminuric insulin-dependent diabetic patients. Hypertension. 1990;15(6 Pt 1):570-575. doi:10.1161/01.hyp.15.6.570 [PubMed: 2347619] [CrossRef]
- 387.
- Jensen JS, Mathiesen ER, Nørgaard K, et al. Increased blood pressure and erythrocyte sodium/lithium countertransport activity are not inherited in diabetic nephropathy. Diabetologia. 1990;33(10):619-624. doi:10.1007/BF00400206 [PubMed: 2257999] [CrossRef]
- 388.
- Carr S, Mbanya JC, Thomas T, et al. Increase in glomerular filtration rate in patients with insulin-dependent diabetes and elevated erythrocyte sodium-lithium countertransport. N Engl J Med. 1990;322(8):500-505. doi:10.1056/NEJM199002223220803 [PubMed: 2300121] [CrossRef]
- 389.
- Lopes de Faria JM, Silveira LA, Morgano M, Pavin EJ, Lopes de Faria JB. Erythrocyte sodium-lithium countertransport and proliferative diabetic retinopathy. Invest Ophthalmol Vis Sci. 2000;41(6):1482-1485. [PubMed: 10798666]
- 390.
- Elving LD, Wetzels JF, de Nobel E, Berden JH. Erythrocyte sodium-lithium countertransport is not different in type 1 (insulin-dependent) diabetic patients with and without diabetic nephropathy. Diabetologia. 1991;34(2):126-128. doi:10.1007/BF00500384 [PubMed: 2065847] [CrossRef]
- 391.
- Gall MA, Rossing P, Jensen JS, Funder J, Parving HH. Red cell Na+/Li+ countertransport in non-insulin-dependent diabetics with diabetic nephropathy. Kidney Int. 1991;39(1):135-140. doi:10.1038/ki.1991.17 [PubMed: 2002627] [CrossRef]
- 392.
- Giordano M, Castellino P, Solini A, Canessa ML, DeFronzo RA. Na+/Li+ and Na+/H+ countertransport activity in hypertensive non-insulin-dependent diabetic patients: role of insulin resistance and antihypertensive treatment. Metabolism. 1997;46(11):1316-1323. doi:10.1016/s0026-0495(97)90237-7 [PubMed: 9361692] [CrossRef]
- 393.
- Fujita J, Tsuda K, Seno M, Obayashi H, Fukui I, Seino Y. Erythrocyte sodium-lithium countertransport activity as a marker of predisposition to hypertension and diabetic nephropathy in NIDDM. Diabetes Care. 1994;17(9):977-982. doi:10.2337/diacare.17.9.977 [PubMed: 7988318] [CrossRef]
- 394.
- Herman WH, Prior DE, Yassine MD, Weder AB. Nephropathy in NIDDM is associated with cellular markers for hypertension. Diabetes Care. 1993;16(5):815-818. doi:10.2337/diacare.16.5.815 [PubMed: 8388330] [CrossRef]
- 395.
- Völzke H, Gruska S, Vogelgesang D, Kerner W, Kraatz G, Rettig R. Intracellular calcium and sodium-lithium countertransport in type 2 diabetic patients with and without albuminuria. Endocr J. 2006;53(6):773-781. doi:10.1507/endocrj.k06-065 [PubMed: 16983181] [CrossRef]
- 396.
- Van Norren K, Thien T, Berden JH, Elving LD, De Pont JJ. Relevance of erythrocyte Na+/Li+ countertransport measurement in essential hypertension, hyperlipidaemia and diabetic nephropathy: a critical review. Eur J Clin Invest. 1998;28(5):339-352. doi:10.1046/j.1365-2362.1998.00302.x [PubMed: 9650006] [CrossRef]
- 397.
- Diamond JR, Karnovsky MJ. Focal and segmental glomerulosclerosis: analogies to atherosclerosis. Kidney Int. 1988;33(5):917-924. doi:10.1038/ki.1988.87 [PubMed: 3292816] [CrossRef]
- 398.
- Moorhead JF, Chan MK, El-Nahas M, Varghese Z. Lipid nephrotoxicity in chronic progressive glomerular and tubulo-interstitial disease. Lancet. 1982;2(8311):1309-1311. doi:10.1016/s0140-6736(82)91513-6 [PubMed: 6128601] [CrossRef]
- 399.
- Cases A, Coll E. Dyslipidemia and the progression of renal disease in chronic renal failure patients. Kidney Int Suppl. 2005;68(99):S87-S93. doi:10.1111/j.1523-1755.2005.09916.x [PubMed: 16336584] [CrossRef]
- 400.
- Marcovecchio ML, Dalton RN, Prevost AT, et al. Prevalence of abnormal lipid profiles and the relationship with the development of microalbuminuria in adolescents with type 1 diabetes. Diabetes Care. 2009;32(4):658-663. doi:10.2337/dc08-1641 [PMC free article: PMC2660471] [PubMed: 19171721] [CrossRef]
- 401.
- Ku E, Campese V. Is lipid management effective for all stages of CKD? Blood Purif. 2013;35(1-3):26-30. doi:10.1159/000345932 [PubMed: 23343543] [CrossRef]
- 402.
- Jenkins AJ, Lyons TJ, Zheng D, et al. Lipoproteins in the DCCT/EDIC cohort: associations with diabetic nephropathy. Kidney Int. 2003;64(3):817-828. doi:10.1046/j.1523-1755.2003.00164.x [PubMed: 12911531] [CrossRef]
- 403.
- Kwan BC, Kronenberg F, Beddhu S, Cheung AK. Lipoprotein metabolism and lipid management in chronic kidney disease. J Am Soc Nephrol. 2007;18(4):1246-1261. doi:10.1681/ASN.2006091006 [PubMed: 17360943] [CrossRef]
- 404.
- Krentz AJ. Lipoprotein abnormalities and their consequences for patients with type 2 diabetes. Diabetes Obes Metab. 2003;5(Suppl 1):S19-S27. doi:10.1046/j.1462-8902.2003.0310.x [PubMed: 14984018] [CrossRef]
- 405.
- Thomas MC, Rosengård-Bärlund M, Mills V, et al. Serum lipids and the progression of nephropathy in type 1 diabetes. Diabetes Care. 2006;29(2):317-322. doi:10.2337/diacare.29.02.06.dc05-0809 [PubMed: 16443880] [CrossRef]
- 406.
- Thorn LM, Forsblom C, Fagerudd J, et al. Metabolic syndrome in type 1 diabetes: association with diabetic nephropathy and glycemic control (the FinnDiane study). Diabetes Care. 2005;28(8):2019-2024. doi:10.2337/diacare.28.8.2019 [PubMed: 16043748] [CrossRef]
- 407.
- Kamanna VS. Low density lipoproteins and mitogenic signal transduction processes: role in the pathogenesis of renal disease. Histol Histopathol. 2002;17(2):497-505. doi:10.14670/HH-17.497 [PubMed: 11962755] [CrossRef]
- 408.
- Sibley SD, Hokanson JE, Steffes MW, et al. Increased small dense LDL and intermediate-density lipoprotein with albuminuria in type 1 diabetes. Diabetes Care. 1999;22(7):1165-1170. doi:10.2337/diacare.22.7.1165 [PMC free article: PMC2635089] [PubMed: 10388983] [CrossRef]
- 409.
- Retnakaran R, Cull CA, Thorne KI, Adler AI, Holman RR, UKPDS Study Group. Risk factors for renal dysfunction in type 2 diabetes: U.K. Prospective Diabetes Study 74. Diabetes. 2006;55(6):1832-1839. doi:10.2337/db05-1620 [PubMed: 16731850] [CrossRef]
- 410.
- Tershakovec AM, Keane WF, Zhang Z, et al. Effect of LDL cholesterol and treatment with losartan on end-stage renal disease in the RENAAL study. Diabetes Care. 2008;31(3):445-447. doi:10.2337/dc07-0196 [PubMed: 18070995] [CrossRef]
- 411.
- Colhoun HM, Lee ET, Bennett PH, et al. Risk factors for renal failure: the WHO Multinational Study of Vascular Disease in Diabetes. Diabetologia. 2001;44(Suppl 2):S46-S53. doi:10.1007/pl00002939 [PubMed: 11587050] [CrossRef]
- 412.
- Rossi MC, Nicolucci A, Pellegrini F, et al. Identifying patients with type 2 diabetes at high risk of microalbuminuria: results of the DEMAND (Developing Education on Microalbuminuria for Awareness of reNal and cardiovascular risk in Diabetes) Study. Nephrol Dial Transplant. 2008;23(4):1278-1284. doi:10.1093/ndt/gfm798 [PubMed: 18039647] [CrossRef]
- 413.
- Smulders YM, Rakic M, Stehouwer CD, Weijers RN, Slaats EH, Silberbusch J. Determinants of progression of microalbuminuria in patients with NIDDM. A prospective study. Diabetes Care. 1997;20(6):999-1005. doi:10.2337/diacare.20.6.999 [PubMed: 9167114] [CrossRef]
- 414.
- Fagot-Campagna A, Nelson RG, Knowler WC, et al. Plasma lipoproteins and the incidence of abnormal excretion of albumin in diabetic American Indians: the Strong Heart Study. Diabetologia. 1998;41(9):1002-1009. doi:10.1007/s001250051023 [PubMed: 9754817] [CrossRef]
- 415.
- Cooper ME, Jandeleit-Dahm KA. Lipids and diabetic renal disease. Curr Diab Rep. 2005;5(6):445-448. doi:10.1007/s11892-005-0053-9 [PubMed: 16316596] [CrossRef]
- 416.
- Gyebi L, Soltani Z, Reisin E. Lipid nephrotoxicity: new concept for an old disease. Curr Hypertens Rep. 2012;14(2):177-181. doi:10.1007/s11906-012-0250-2 [PubMed: 22290079] [CrossRef]
- 417.
- Attia DM, Feron O, Goldschmeding R, et al. Hypercholesterolemia in rats induces podocyte stress and decreases renal cortical nitric oxide synthesis via an angiotensin II type 1 receptor-sensitive mechanism. J Am Soc Nephrol. 2004;15(4):949-957. doi:10.1097/01.asn.0000118528.00817.8e [PubMed: 15034097] [CrossRef]
- 418.
- Attia DM, Ni ZN, Boer P, et al. Proteinuria is preceded by decreased nitric oxide synthesis and prevented by a NO donor in cholesterol-fed rats. Kidney Int. 2002;61(5):1776-1787. doi:10.1046/j.1523-1755.2002.00313.x [PubMed: 11967027] [CrossRef]
- 419.
- Chade AR, Zhu XY, Grande JP, Krier JD, Lerman A, Lerman LO. Simvastatin abates development of renal fibrosis in experimental renovascular disease. J Hypertens. 2008;26(8):1651-1660. doi:10.1097/HJH.0b013e328302833a [PubMed: 18622245] [CrossRef]
- 420.
- Feron O, Dessy C, Moniotte S, Desager JP, Balligand JL. Hypercholesterolemia decreases nitric oxide production by promoting the interaction of caveolin and endothelial nitric oxide synthase. J Clin Invest. 1999;103(6):897-905. doi:10.1172/JCI4829 [PMC free article: PMC408139] [PubMed: 10079111] [CrossRef]
- 421.
- Romero JC, Reckelhoff JF. State-of-the-art lecture. Role of angiotensin and oxidative stress in essential hypertension. Hypertension. 1999;34(4 Pt 2):943-949. doi:10.1161/01.hyp.34.4.943 [PubMed: 10523389] [CrossRef]
- 422.
- Kopkan L, Khan MAH, Lis A, Awayda MS, Majid DS. Cholesterol induces renal vasoconstriction and anti-natriuresis by inhibiting nitric oxide production in anesthetized rats. Am J Physiol Renal Physiol. 2009;297(6):F1606-F1613. doi:10.1152/ajprenal.90743.2008 [PMC free article: PMC2801331] [PubMed: 19776170] [CrossRef]
- 423.
- Nosadini R, Tonolo G. Role of oxidized low density lipoproteins and free fatty acids in the pathogenesis of glomerulopathy and tubulointerstitial lesions in type 2 diabetes. Nutr Metab Cardiovasc Dis. 2011;21(2):79-85. doi:10.1016/j.numecd.2010.10.002 [PubMed: 21186102] [CrossRef]
- 424.
- Tang C, Kanter JE, Bornfeldt KE, Leboeuf RC, Oram JF. Diabetes reduces the cholesterol exporter ABCA1 in mouse macrophages and kidneys. J Lipid Res. 2010;51(7):1719-1728. doi:10.1194/jlr.M003525 [PMC free article: PMC2882721] [PubMed: 19965614] [CrossRef]
- 425.
- Proctor G, Jiang T, Iwahashi M, Wang Z, Li J, Levi M. Regulation of renal fatty acid and cholesterol metabolism, inflammation, and fibrosis in Akita and OVE26 mice with type 1 diabetes. Diabetes. 2006;55(9):2502-2509. doi:10.2337/db05-0603 [PubMed: 16936198] [CrossRef]
- 426.
- Merscher-Gomez S, Guzman J, Pedigo CE, et al. Cyclodextrin protects podocytes in diabetic kidney disease. Diabetes. 2013;62(11):3817-3827. doi:10.2337/db13-0399 [PMC free article: PMC3806621] [PubMed: 23835338] [CrossRef]
- 427.
- Herman-Edelstein M, Scherzer P, Tobar A, Levi M, Gafter U. Altered renal lipid metabolism and renal lipid accumulation in human diabetic nephropathy. J Lipid Res. 2014;55(3):561-572. doi:10.1194/jlr.P040501 [PMC free article: PMC3934740] [PubMed: 24371263] [CrossRef]
- 428.
- Mittendorfer B, Klein S, Fontana L. A word of caution against excessive protein intake. Nat Rev Endocrinol. 2020;16(1):59-66. doi:10.1038/s41574-019-0274-7 [PubMed: 31728051] [CrossRef]
- 429.
- van Nielen M, Feskens EJ, Mensink M, et al. Dietary protein intake and incidence of type 2 diabetes in Europe: the EPIC-InterAct Case-Cohort Study. Diabetes Care. 2014;37(7):1854-1862. doi:10.2337/dc13-2627 [PubMed: 24722499] [CrossRef]
- 430.
- Hoogeveen EK, Kostense PJ, Jager A, et al. Serum homocysteine level and protein intake are related to risk of microalbuminuria: the Hoorn Study. Kidney Int. 1998;54(1):203-209. doi:10.1038/sj.ki.4495353 [PubMed: 9648080] [CrossRef]
- 431.
- Farr LE, Smadel JE. The effect of dietary protein on the course of nephrotoxic nephritis in rats. J Exp Med. 1939;70(6):615-627. doi:10.1084/jem.70.6.615 [PMC free article: PMC2133771] [PubMed: 19870934] [CrossRef]
- 432.
- Friend PS, Fernandes G, Good RA, Michael AF, Yunis EJ. Dietary restrictions early and late: effects on the nephropathy of the NZB X NZW mouse. Lab Invest. 1978;38(6):629-632. [PubMed: 96299]
- 433.
- Hostetter TH, Olson JL, Rennke HG, Venkatachalam MA, Brenner BM. Hyperfiltration in remnant nephrons: a potentially adverse response to renal ablation. Am J Physiol. 1981;241(1):F85-F93. doi:10.1152/ajprenal.1981.241.1.F85 [PubMed: 7246778] [CrossRef]
- 434.
- Hostetter TH, Meyer TW, Rennke HG, Brenner BM. Chronic effects of dietary protein in the rat with intact and reduced renal mass. Kidney Int. 1986;30(4):509-517. doi:10.1038/ki.1986.215 [PubMed: 3784291] [CrossRef]
- 435.
- Zatz R, Meyer TW, Rennke HG, Brenner BM. Predominance of hemodynamic rather than metabolic factors in the pathogenesis of diabetic glomerulopathy. Proc Natl Acad Sci U S A. 1985;82(17):5963-5967. doi:10.1073/pnas.82.17.5963 [PMC free article: PMC390674] [PubMed: 3862110] [CrossRef]
- 436.
- Meek RL, LeBoeuf RC, Saha SA, et al. Glomerular cell death and inflammation with high-protein diet and diabetes. Nephrol Dial Transplant. 2013;28(7):1711-1720. doi:10.1093/ndt/gfs579 [PMC free article: PMC3707525] [PubMed: 23314315] [CrossRef]
- 437.
- Tuttle KR, Bruton JL, Perusek MC, Lancaster JL, Kopp DT, DeFronzo RA. Effect of strict glycemic control on renal hemodynamic response to amino acids and renal enlargement in insulin-dependent diabetes mellitus. N Engl J Med. 1991;324(23):1626-1632. doi:10.1056/NEJM199106063242304 [PubMed: 2030719] [CrossRef]
- 438.
- Tuttle KR, Bruton JL. Effect of insulin therapy on renal hemodynamic response to amino acids and renal hypertrophy in non-insulin-dependent diabetes. Kidney Int. 1992;42(1):167-173. doi:10.1038/ki.1992.274 [PubMed: 1635346] [CrossRef]
- 439.
- Uribarri J, Tuttle KR. Advanced glycation end products and nephrotoxicity of high-protein diets. Clin J Am Soc Nephrol. 2006;1(6):1293-1299. doi:10.2215/CJN.01270406 [PubMed: 17699361] [CrossRef]
- 440.
- Banerjee T, Crews DC, Wesson DE, et al. Dietary acid load and chronic kidney disease among adults in the United States. BMC Nephrol. 2014;15:137. doi:10.1186/1471-2369-15-137 [PMC free article: PMC4151375] [PubMed: 25151260] [CrossRef]
- 441.
- Shah SN, Abramowitz M, Hostetter TH, Melamed ML. Serum bicarbonate levels and the progression of kidney disease: a cohort study. Am J Kidney Dis. 2009;54(2):270-277. doi:10.1053/j.ajkd.2009.02.014 [PMC free article: PMC4354889] [PubMed: 19394734] [CrossRef]
- 442.
- Banerjee T, McCulloch CE, Crews DC, et al. Proinflammatory diets and risk of ESKD in US adults with CKD. Kidney360. 2022;3(11):1852-1860. doi:10.34067/KID.0000442022 [PMC free article: PMC9717620] [PubMed: 36514411] [CrossRef]
- 443.
- Whaley-Connell AT, Sowers JR, Stevens LA, et al. CKD in the United States: Kidney Early Evaluation Program (KEEP) and National Health and Nutrition Examination Survey (NHANES) 1999–2004. Am J Kidney Dis. 2008;51(4 Suppl 2):S13-S20. doi:10.1053/j.ajkd.2007.12.016 [PubMed: 18359403] [CrossRef]
- 444.
- Rudberg S, Stattin EL, Dahlquist G. Familial and perinatal risk factors for micro- and macroalbuminuria in young IDDM patients. Diabetes. 1998;47(7):1121-1126. doi:10.2337/diabetes.47.7.1121 [PubMed: 9648837] [CrossRef]
- 445.
- Chase HP, Garg SK, Marshall G, et al. Cigarette smoking increases the risk of albuminuria among subjects with type I diabetes. JAMA. 1991;265(5):614-617. doi:10.1001/jama.1991.03460050068022 [PubMed: 1987411] [CrossRef]
- 446.
- Mühlhauser I, Sawicki P, Berger M. Cigarette-smoking as a risk factor for macroproteinuria and proliferative retinopathy in type 1 (insulin-dependent) diabetes. Diabetologia. 1986;29(8):500-502. doi:10.1007/BF00453501 [PubMed: 3758532] [CrossRef]
- 447.
- Hovind P, Rossing P, Tarnow L, Parving HH. Smoking and progression of diabetic nephropathy in type 1 diabetes. Diabetes Care. 2003;26(3):911-916. doi:10.2337/diacare.26.3.911 [PubMed: 12610058] [CrossRef]
- 448.
- Mühlhauser I, Bender R, Bott U, et al. Cigarette smoking and progression of retinopathy and nephropathy in type 1 diabetes. Diabet Med. 1996;13(6):536-543. doi:10.1002/(SICI)1096-9136(199606)13:6<536::AID-DIA110>3.0.CO;2-J [PubMed: 8799657] [CrossRef]
- 449.
- Biesenbach G, Janko O, Zazgornik J. Similar rate of progression in the predialysis phase in type I and type II diabetes mellitus. Nephrol Dial Transplant. 1994;9(8):1097-1102. doi:10.1093/ndt/9.8.1097 [PubMed: 7800207] [CrossRef]
- 450.
- Sawicki PT, Didjurgeit U, Mühlhauser I, Bender R, Heinemann L, Berger M. Smoking is associated with progression of diabetic nephropathy. Diabetes Care. 1994;17(2):126-131. doi:10.2337/diacare.17.2.126 [PubMed: 8137682] [CrossRef]
- 451.
- Hsu CC, Hwang SJ, Tai TY, et al. Cigarette smoking and proteinuria in Taiwanese men with type 2 diabetes mellitus. Diabet Med. 2010;27(3):295-302. doi:10.1111/j.1464-5491.2010.02947.x [PubMed: 20536492] [CrossRef]
- 452.
- Chuahirun T, Khanna A, Kimball K, Wesson DE. Cigarette smoking and increased urine albumin excretion are interrelated predictors of nephropathy progression in type 2 diabetes. Am J Kidney Dis. 2003;41(1):13-21. doi:10.1053/ajkd.2003.50009 [PubMed: 12500217] [CrossRef]
- 453.
- Gambaro G, Bax G, Fusaro M, et al. Cigarette smoking is a risk factor for nephropathy and its progression in type 2 diabetes mellitus. Diabetes Nutr Metab. 2001;14(6):337-342. [PubMed: 11853366]
- 454.
- Afghahi H, Cederholm J, Eliasson B, et al. Risk factors for the development of albuminuria and renal impairment in type 2 diabetes—the Swedish National Diabetes Register (NDR). Nephrol Dial Transplant. 2011;26(4):1236-1243. doi:10.1093/ndt/gfq535 [PubMed: 20817668] [CrossRef]
- 455.
- Huang MF, Lin WL, Ma YC. A study of reactive oxygen species in mainstream of cigarette. Indoor Air. 2005;15(2):135-140. doi:10.1111/j.1600-0668.2005.00330.x [PubMed: 15737156] [CrossRef]
- 456.
- Wilkes HC, Kelleher C, Meade TW. Smoking and plasma fibrinogen. Lancet. 1988;1(8580):307-308. doi:10.1016/s0140-6736(88)90398-4 [PubMed: 2893128] [CrossRef]
- 457.
- Paterson AM, Mancia G, FitzGerald GA. Proceedings of the Symposium on Smoking: a Risk Factor for Cardiovascular Disease. Am Heart J. 1988;115(Suppl 2):S240-S294.
- 458.
- Deckert T, Feldt-Rasmussen B, Borch-Johnsen K, Jensen T, Kofoed-Enevoldsen A. Albuminuria reflects widespread vascular damage. The Steno hypothesis. Diabetologia. 1989;32(4):219-226. doi:10.1007/BF00285287 [PubMed: 2668076] [CrossRef]
- 459.
- Ogden CL, Flegal KM, Carroll MD, Johnson CL. Prevalence and trends in overweight among US children and adolescents, 1999–2000. JAMA. 2002;288(14):1728-1732. doi:10.1001/jama.288.14.1728 [PubMed: 12365956] [CrossRef]
- 460.
- Nelson RG, Pavkov ME, Hanson RL, Knowler WC. Changing course of diabetic nephropathy in the Pima Indians. Diabetes Res Clin Pract. 2008;82(Suppl 1):S10-S14. doi:10.1016/j.diabres.2008.09.014 [PMC free article: PMC2603306] [PubMed: 18842316] [CrossRef]
- 461.
- Yarnoff BO, Hoerger TJ, Shrestha SS, et al. Modeling the impact of obesity on the lifetime risk of chronic kidney disease in the United States using updated estimates of GFR progression from the CRIC study. PLoS One. 2018;13(10):e0205530. doi:10.1371/journal.pone.0205530 [PMC free article: PMC6195263] [PubMed: 30339684] [CrossRef]
- 462.
- Pavkov ME, Hanson RL, Knowler WC, Bennett PH, Krakoff J, Nelson RG. Changing patterns of type 2 diabetes incidence among Pima Indians. Diabetes Care. 2007;30(7):1758-1763. doi:10.2337/dc06-2010 [PubMed: 17468358] [CrossRef]
- 463.
- Wang Y, Chen X, Song Y, Caballero B, Cheskin LJ. Association between obesity and kidney disease: a systematic review and meta-analysis. Kidney Int. 2008;73(1):19-33. doi:10.1038/sj.ki.5002586 [PubMed: 17928825] [CrossRef]
- 464.
- Kambham N, Markowitz GS, Valeri AM, Lin J, D’Agati VD. Obesity-related glomerulopathy: an emerging epidemic. Kidney Int. 2001;59(4):1498-1509. doi:10.1046/j.1523-1755.2001.0590041498.x [PubMed: 11260414] [CrossRef]
- 465.
- Taylor GW, Borgnakke WS. Periodontal disease: associations with diabetes, glycemic control and complications. Oral Dis. 2008;14(3):191-203. doi:10.1111/j.1601-0825.2008.01442.x [PubMed: 18336370] [CrossRef]
- 466.
- Akar H, Akar GC, Carrero JJ, Stenvinkel P, Lindholm B. Systemic consequences of poor oral health in chronic kidney disease patients. Clin J Am Soc Nephrol. 2011;6(1):218-226. doi:10.2215/CJN.05470610 [PubMed: 21115624] [CrossRef]
- 467.
- Xiong X, Buekens P, Vastardis S, Pridjian G. Periodontal disease and gestational diabetes mellitus. Am J Obstet Gynecol. 2006;195(4):1086-1089. doi:10.1016/j.ajog.2006.06.035 [PubMed: 16846573] [CrossRef]
- 468.
- Kshirsagar AV, Craig RG, Moss KL, et al. Periodontal disease adversely affects the survival of patients with end-stage renal disease. Kidney Int. 2009;75(7):746-751. doi:10.1038/ki.2008.660 [PubMed: 19165177] [CrossRef]
- 469.
- Shultis WA, Weil EJ, Looker HC, et al. Effect of periodontitis on overt nephropathy and end-stage renal disease in type 2 diabetes. Diabetes Care. 2007;30(2):306-311. doi:10.2337/dc06-1184 [PubMed: 17259499] [CrossRef]
- 470.
- Fisher MA, Taylor GW, West BT, McCarthy ET. Bidirectional relationship between chronic kidney disease and periodontal disease: structural equation modeling. Kidney Int. 2011;79(3):347-355. doi:10.1038/ki.2010.384 [PMC free article: PMC3045269] [PubMed: 20927035] [CrossRef]
- 471.
- Sanz M, D’Aiuto F, Deanfield J, Fernandez-Avilés F. European workshop in periodontal health and cardiovascular disease—scientific evidence on the association between periodontal and cardiovascular diseases: a review of the literature. Eur Heart J Suppl. 2010;12(Suppl B):B3-B12. doi:10.1093/eurheartj/suq003 [CrossRef]
- 472.
- Tonetti MS, D’Aiuto F, Nibali L, et al. Treatment of periodontitis and endothelial function. N Engl J Med. 2007;356(9):911-920. doi:10.1056/NEJMoa063186 [PubMed: 17329698] [CrossRef]
- 473.
- Harirforoosh S, Jamali F. Renal adverse effects of nonsteroidal anti-inflammatory drugs. Expert Opin Drug Saf. 2009;8(6):669-681. doi:10.1517/14740330903311023 [PubMed: 19832117] [CrossRef]
- 474.
- Evans M, Fored CM, Bellocco R, et al. Acetaminophen, aspirin and progression of advanced chronic kidney disease. Nephrol Dial Transplant. 2009;24(6):1908-1918. doi:10.1093/ndt/gfn745 [PubMed: 19155536] [CrossRef]
- 475.
- Musu M, Finco G, Antonucci R, et al. Acute nephrotoxicity of NSAID from the foetus to the adult. Eur Rev Med Pharmacol Sci. 2011;15(12):1461-1472. [PubMed: 22288307]
- 476.
- Adams DH, Howie AJ, Michael J, McConkey B, Bacon PA, Adu D. Non-steroidal anti-inflammatory drugs and renal failure. Lancet. 1986;1(8472):57-60. doi:10.1016/s0140-6736(86)90714-2 [PubMed: 2867313] [CrossRef]
- 477.
- Perneger TV, Whelton PK, Klag MJ. Risk of kidney failure associated with the use of acetaminophen, aspirin, and nonsteroidal antiinflammatory drugs. N Engl J Med. 1994;331(25):1675-1679. doi:10.1056/NEJM199412223312502 [PubMed: 7969358] [CrossRef]
- 478.
- Radhakrishnan J, Perazella MA. Drug-induced glomerular disease: attention required! Clin J Am Soc Nephrol. 2015;10(7):1287-1290. doi:10.2215/CJN.01010115 [PMC free article: PMC4491281] [PubMed: 25876771] [CrossRef]
- 479.
- Markowitz GS, Bomback AS, Perazella MA. Drug-induced glomerular disease: direct cellular injury. Clin J Am Soc Nephrol. 2015;10(7):1291-1299. doi:10.2215/CJN.00860115 [PMC free article: PMC4491280] [PubMed: 25862776] [CrossRef]
- 480.
- McCullough PA, Adam A, Becker CR, et al. Risk prediction of contrast-induced nephropathy. Am J Cardiol. 2006;98(6A):27K-36K. doi:10.1016/j.amjcard.2006.01.022 [PubMed: 16949378] [CrossRef]
- 481.
- Koopman RJ, Mainous AG, 3rd, Liszka HA, et al. Evidence of nephropathy and peripheral neuropathy in US adults with undiagnosed diabetes. Ann Fam Med. 2006;4(5):427-432. doi:10.1370/afm.577 [PMC free article: PMC1578655] [PubMed: 17003143] [CrossRef]
- 482.
- Sundkvist G, Lilja B. Autonomic neuropathy predicts deterioration in glomerular filtration rate in patients with IDDM. Diabetes Care. 1993;16(5):773-779. doi:10.2337/diacare.16.5.773 [PubMed: 8495619] [CrossRef]
- 483.
- Lilja B, Nosslin B, Bergström B, Sundkvist G. Glomerular filtration rate, autonomic nerve function, and orthostatic blood pressure in patients with diabetes mellitus. Diabetes Res. 1985;2(4):179-181. [PubMed: 4085171]
- 484.
- Kim YK, Lee JE, Kim YG, et al. Cardiac autonomic neuropathy as a predictor of deterioration of the renal function in normoalbuminuric, normotensive patients with type 2 diabetes mellitus. J Korean Med Sci. 2009;24(Suppl 1):S69-S74. doi:10.3346/jkms.2009.24.S1.S69 [PMC free article: PMC2633204] [PubMed: 19194565] [CrossRef]
- 485.
- Ewing DJ, Campbell IW, Clarke BF. The natural history of diabetic autonomic neuropathy. Q J Med. 1980;49(1):95-108. doi:10.1093/oxfordjournals.qjmed.a067610 [PubMed: 7433630] [CrossRef]
- 486.
- Bramham K, Rajasingham D. Pregnancy in diabetes and kidney disease. J Ren Care. 2012;38(Suppl 1):78-89. doi:10.1111/j.1755-6686.2012.00270.x [PubMed: 22348367] [CrossRef]
- 487.
- Biesenbach G, Stöger W, Zazgornik J. [Changes in renal protein excretion and kidney function in type I diabetic patients during and following pregnancy in relation to the stage of preexistent diabetic nephropathy] [Article in German]. Klin Wochenschr 1987;65(21):1048–1053. doi:10.1007/BF01726324 [PubMed: 3431031] [CrossRef]
- 488.
- Powe CE, Thadhani R. Diabetes and the kidney in pregnancy. Semin Nephrol. 2011;31(1):59-69. doi:10.1016/j.semnephrol.2010.10.006 [PubMed: 21266265] [CrossRef]
- 489.
- Diabetes Control Complications Trial Research Group. Effect of pregnancy on microvascular complications in the Diabetes Control and Complications Trial. Diabetes Care. 2000;23(8):1084-1091. doi:10.2337/diacare.23.8.1084 [PMC free article: PMC2631985] [PubMed: 10937502] [CrossRef]
- 490.
- Gordon M, Landon MB, Samuels P, Hissrich S, Gabbe SG. Perinatal outcome and long-term follow-up associated with modern management of diabetic nephropathy. Obstet Gynecol. 1996;87(3):401-409. doi:10.1016/0029-7844(95)00420-3 [PubMed: 8598963] [CrossRef]
- 491.
- Rossing K, Jacobsen P, Hommel E, et al. Pregnancy and progression of diabetic nephropathy. Diabetologia. 2002;45(1):36-41. doi:10.1007/s125-002-8242-4 [PubMed: 11845221] [CrossRef]
- 492.
- Gordin D, Hiilesmaa V, Fagerudd J, et al. Pre-eclampsia but not pregnancy-induced hypertension is a risk factor for diabetic nephropathy in type 1 diabetic women. Diabetologia. 2007;50(3):516-522. doi:10.1007/s00125-006-0544-5 [PubMed: 17216281] [CrossRef]
- 493.
- Rocco L, Gil FZ, da Fonseca Pletiskaitz TM, de Fátima Cavanal M, Gomes GN. Effect of sodium overload on renal function of offspring from diabetic mothers. Pediatr Nephrol. 2008;23(11):2053-2060. doi:10.1007/s00467-008-0884-0 [PubMed: 18574600] [CrossRef]
- 494.
- Nelson RG. Intrauterine determinants of diabetic kidney disease in disadvantaged populations. Kidney Int Suppl. 2003;63(83):S13-S16. doi:10.1046/j.1523-1755.63.s83.4.x [PubMed: 12864868] [CrossRef]
- 495.
- Luyckx VA, Bertram JF, Brenner BM, et al. Effect of fetal and child health on kidney development and long-term risk of hypertension and kidney disease. Lancet. 2013;382(9888):273-283. doi:10.1016/S0140-6736(13)60311-6 [PubMed: 23727166] [CrossRef]
- 496.
- Ruta LAM, Dickinson H, Thomas MC, Denton KM, Anderson WP, Kett MM. High-salt diet reveals the hypertensive and renal effects of reduced nephron endowment. Am J Physiol Renal Physiol. 2010;298(6):F1384-F1392. doi:10.1152/ajprenal.00049.2010 [PubMed: 20335316] [CrossRef]
- 497.
- Pinheiro AR, Salvucci ID, Aguila MB, Mandarim-de-Lacerda CA. Protein restriction during gestation and/or lactation causes adverse transgenerational effects on biometry and glucose metabolism in F1 and F2 progenies of rats. Clin Sci (Lond). 2008;114(5):381-392. doi:10.1042/CS20070302 [PubMed: 17927565] [CrossRef]
- 498.
- Harrison M, Langley-Evans SC. Intergenerational programming of impaired nephrogenesis and hypertension in rats following maternal protein restriction during pregnancy. Br J Nutr. 2009;101(7):1020-1030. doi:10.1017/S0007114508057607 [PMC free article: PMC2665257] [PubMed: 18778527] [CrossRef]
- 499.
- Reddy MA, Natarajan R. Epigenetics in diabetic kidney disease. J Am Soc Nephrol. 2011;22(12):2182-2185. doi:10.1681/ASN.2011060629 [PMC free article: PMC3250203] [PubMed: 22021712] [CrossRef]
- 500.
- Dabelea D, Hanson RL, Bennett PH, Roumain J, Knowler WC, Pettitt DJ. Increasing prevalence of type II diabetes in American Indian children. Diabetologia. 1998;41(8):904-910. doi:10.1007/s001250051006 [PubMed: 9726592] [CrossRef]
- 501.
- Abi Khalil C, Travert F, Fetita S, et al. Fetal exposure to maternal type 1 diabetes is associated with renal dysfunction at adult age. Diabetes. 2010;59(10):2631-2636. doi:10.2337/db10-0419 [PMC free article: PMC3279566] [PubMed: 20622173] [CrossRef]
- 502.
- Abrahamson DR, Steenhard BM. Perinatal nephron programming is not so sweet in maternal diabetes. J Am Soc Nephrol. 2008;19(5):837-839. doi:10.1681/ASN.2008030280 [PubMed: 18385416] [CrossRef]
- 503.
- Tran S, Chen YW, Chenier I, et al. Maternal diabetes modulates renal morphogenesis in offspring. J Am Soc Nephrol. 2008;19(5):943-952. doi:10.1681/ASN.2007080864 [PMC free article: PMC2386724] [PubMed: 18305124] [CrossRef]
- 504.
- White SL, Perkovic V, Cass A, et al. Is low birth weight an antecedent of CKD in later life? A systematic review of observational studies. Am J Kidney Dis. 2009;54(2):248-261. doi:10.1053/j.ajkd.2008.12.042 [PubMed: 19339091] [CrossRef]
- 505.
- Li S, Chen SC, Shlipak M, et al. Low birth weight is associated with chronic kidney disease only in men. Kidney Int. 2008;73(5):637-642. doi:10.1038/sj.ki.5002747 [PubMed: 18094674] [CrossRef]
- 506.
- Hsu CW, Yamamoto KT, Henry RK, De Roos AJ, Flynn JT. Prenatal risk factors for childhood CKD. J Am Soc Nephrol. 2014;25(9):2105-2111. doi:10.1681/ASN.2013060582 [PMC free article: PMC4147970] [PubMed: 24744441] [CrossRef]
- 507.
- Seaquist ER, Goetz FC, Rich S, Barbosa J. Familial clustering of diabetic kidney disease. Evidence for genetic susceptibility to diabetic nephropathy. N Engl J Med. 1989;320(18):1161-1165. doi:10.1056/NEJM198905043201801 [PubMed: 2710189] [CrossRef]
- 508.
- Borch-Johnsen K, Nørgaard K, Hommel E, et al. Is diabetic nephropathy an inherited complication? Kidney Int. 1992;41(4):719-722. doi:10.1038/ki.1992.112 [PubMed: 1513092] [CrossRef]
- 509.
- Pettitt DJ, Saad MF, Bennett PH, Nelson RG, Knowler WC. Familial predisposition to renal disease in two generations of Pima Indians with type 2 (non-insulin-dependent) diabetes mellitus. Diabetologia. 1990;33(7):438-443. doi:10.1007/BF00404096 [PubMed: 2401399] [CrossRef]
- 510.
- Hanson RL, Craig DW, Millis MP, et al. Identification of PVT1 as a candidate gene for end-stage renal disease in type 2 diabetes using a pooling-based genome-wide single nucleotide polymorphism association study. Diabetes. 2007;56(4):975-983. doi:10.2337/db06-1072 [PubMed: 17395743] [CrossRef]
- 511.
- Millis MP, Bowen D, Kingsley C, Watanabe RM, Wolford JK. Variants in the plasmacytoma variant translocation gene (PVT1) are associated with end-stage renal disease attributed to type 1 diabetes. Diabetes. 2007;56(12):3027-3032. doi:10.2337/db07-0675 [PubMed: 17881614] [CrossRef]
- 512.
- Pezzolesi MG, Poznik GD, Mychaleckyj JC, et al. Genome-wide association scan for diabetic nephropathy susceptibility genes in type 1 diabetes. Diabetes. 2009;58(6):1403-1410. doi:10.2337/db08-1514 [PMC free article: PMC2682673] [PubMed: 19252134] [CrossRef]
- 513.
- Writing Team for the Diabetes Control and Complications Trial/Epidemiology of Diabetes Interventions and Complications Research Group. Sustained effect of intensive treatment of type 1 diabetes mellitus on development and progression of diabetic nephropathy: the Epidemiology of Diabetes Interventions and Complications (EDIC) study. JAMA. 2003;290(16):2159-2167. doi:10.1001/jama.290.16.2159 [PMC free article: PMC2622725] [PubMed: 14570951] [CrossRef]
- 514.
- Pezzolesi MG, Poznik GD, Skupien J, et al. An intergenic region on chromosome 13q33.3 is associated with the susceptibility to kidney disease in type 1 and 2 diabetes. Kidney Int. 2011;80(1):105-111. doi:10.1038/ki.2011.64 [PMC free article: PMC3774030] [PubMed: 21412220] [CrossRef]
- 515.
- Craig DW, Millis MP, DiStefano JK. Genome-wide SNP genotyping study using pooled DNA to identify candidate markers mediating susceptibility to end-stage renal disease attributed to type 1 diabetes. Diabet Med. 2009;26(11):1090-1098. doi:10.1111/j.1464-5491.2009.02846.x [PubMed: 19929986] [CrossRef]
- 516.
- Shimazaki A, Kawamura Y, Kanazawa A, et al. Genetic variations in the gene encoding ELMO1 are associated with susceptibility to diabetic nephropathy. Diabetes. 2005;54(4):1171-1178. doi:10.2337/diabetes.54.4.1171 [PubMed: 15793258] [CrossRef]
- 517.
- Leak TS, Perlegas PS, Smith SG, et al. Variants in intron 13 of the ELMO1 gene are associated with diabetic nephropathy in African Americans. Ann Hum Genet. 2009;73(2):152-159. doi:10.1111/j.1469-1809.2008.00498.x [PMC free article: PMC2778056] [PubMed: 19183347] [CrossRef]
- 518.
- Knowler WC, Coresh J, Elston RC, et al. The Family Investigation of Nephropathy and Diabetes (FIND): design and methods. J Diabetes Complications. 2005;19(1):1-9. doi:10.1016/j.jdiacomp.2003.12.007 [PubMed: 15642484] [CrossRef]
- 519.
- Iyengar SK, Abboud HE, Goddard KA, et al. Genome-wide scans for diabetic nephropathy and albuminuria in multiethnic populations: the Family Investigation of Nephropathy and Diabetes (FIND). Diabetes. 2007;56(6):1577-1585. doi:10.2337/db06-1154 [PubMed: 17363742] [CrossRef]
- 520.
- Schelling JR, Abboud HE, Nicholas SB, et al. Genome-wide scan for estimated glomerular filtration rate in multi-ethnic diabetic populations: the Family Investigation of Nephropathy and Diabetes (FIND). Diabetes. 2008;57(1):235-243. doi:10.2337/db07-0313 [PubMed: 18003762] [CrossRef]
- 521.
- Mooyaart AL, Valk EJ, van Es LA, et al. Genetic associations in diabetic nephropathy: a meta-analysis. Diabetologia. 2011;54(3):544-553. doi:10.1007/s00125-010-1996-1 [PMC free article: PMC3034040] [PubMed: 21127830] [CrossRef]
- 522.
- Sandholm N, Cole JB, Nair V, et al. Genome-wide meta-analysis and omics integration identifies novel genes associated with diabetic kidney disease. Diabetologia. 2022;65(9):1495-1509. doi:10.1007/s00125-022-05735-0 [PMC free article: PMC9345823] [PubMed: 35763030] [CrossRef]
- 523.
- Langefeld CD, Beck SR, Bowden DW, Rich SS, Wagenknecht LE, Freedman BI. Heritability of GFR and albuminuria in Caucasians with type 2 diabetes mellitus. Am J Kidney Dis. 2004;43(5):796-800. doi:10.1053/j.ajkd.2003.12.043 [PubMed: 15112169] [CrossRef]
- 524.
- Reddy MA, Zhang E, Natarajan R. Epigenetic mechanisms in diabetic complications and metabolic memory. Diabetologia. 2015;58(3):443-455. doi:10.1007/s00125-014-3462-y [PMC free article: PMC4324095] [PubMed: 25481708] [CrossRef]
- 525.
- Alvarez ML, DiStefano JK. The role of non-coding RNAs in diabetic nephropathy: potential applications as biomarkers for disease development and progression. Diabetes Res Clin Pract. 2013;99(1):1-11. doi:10.1016/j.diabres.2012.10.010 [PubMed: 23102915] [CrossRef]
- 526.
- Dwivedi RS, Herman JG, McCaffrey TA, Raj DS. Beyond genetics: epigenetic code in chronic kidney disease. Kidney Int. 2011;79(1):23-32. doi:10.1038/ki.2010.335 [PMC free article: PMC3867804] [PubMed: 20881938] [CrossRef]
- 527.
- Kroc Collaborative Study Group. Blood glucose control and the evolution of diabetic retinopathy and albuminuria. A preliminary multicenter trial. N Engl J Med. 1984;311(6):365-372. doi:10.1056/NEJM198408093110604 [PubMed: 6377076] [CrossRef]
- 528.
- Bending JJ, Viberti GC, Watkins PJ, Keen H. Intermittent clinical proteinuria and renal function in diabetes: evolution and the effect of glycaemic control. Br Med J (Clin Res Ed). 1986;292(6513):83-86. doi:10.1136/bmj.292.6513.83 [PMC free article: PMC1339106] [PubMed: 3080101] [CrossRef]
- 529.
- Feldt-Rasmussen B, Mathiesen ER, Deckert T. Effect of two years of strict metabolic control on progression of incipient nephropathy in insulin-dependent diabetes. Lancet. 1986;2(8519):1300-1304. doi:10.1016/s0140-6736(86)91433-9 [PubMed: 2878175] [CrossRef]
- 530.
- Dahl-Jørgensen K, Bjøro T, Kierulf P, Sandvik L, Bangstad HJ, Hanssen KF. Long-term glycemic control and kidney function in insulin-dependent diabetes mellitus. Kidney Int. 1992;41(4):920-923. doi:10.1038/ki.1992.140 [PubMed: 1513113] [CrossRef]
- 531.
- Reichard P, Nilsson BY, Rosenqvist U. The effect of long-term intensified insulin treatment on the development of microvascular complications of diabetes mellitus. N Engl J Med. 1993;329(5):304-309. doi:10.1056/NEJM199307293290502 [PubMed: 8147960] [CrossRef]
- 532.
- DCCT/EDIC Research Group, de Boer IH, Sun W, et al. Intensive diabetes therapy and glomerular filtration rate in type 1 diabetes. N Engl J Med. 2011;365(25):2366-2376. doi:10.1056/NEJMoa1111732 [PMC free article: PMC3270008] [PubMed: 22077236] [CrossRef]
- 533.
- Nathan DM, Cleary PA, Backlund JY, et al. Intensive diabetes treatment and cardiovascular disease in patients with type 1 diabetes. N Engl J Med. 2005;353(25):2643-2653. doi:10.1056/NEJMoa052187 [PMC free article: PMC2637991] [PubMed: 16371630] [CrossRef]
- 534.
- Holman RR, Paul SK, Bethel MA, Matthews DR, Neil HA. 10-year follow-up of intensive glucose control in type 2 diabetes. N Engl J Med. 2008;359(15):1577-1589. doi:10.1056/NEJMoa0806470 [PubMed: 18784090] [CrossRef]
- 535.
- Del Prato S. Megatrials in type 2 diabetes. From excitement to frustration? Diabetologia. 2009;52(7):1219-1226. doi:10.1007/s00125-009-1352-5 [PubMed: 19373446] [CrossRef]
- 536.
- Duckworth WC, Abraira C, Moritz TE, et al. The duration of diabetes affects the response to intensive glucose control in type 2 subjects: the VA Diabetes Trial. J Diabetes Complications. 2011;25(6):355-361. doi:10.1016/j.jdiacomp.2011.10.003 [PubMed: 22055259] [CrossRef]
- 537.
- Boussageon R, Bejan-Angoulvant T, Saadatian-Elahi M, et al. Effect of intensive glucose lowering treatment on all cause mortality, cardiovascular death, and microvascular events in type 2 diabetes: meta-analysis of randomised controlled trials. BMJ. 2011;343:d4169. doi:10.1136/bmj.d4169 [PMC free article: PMC3144314] [PubMed: 21791495] [CrossRef]
- 538.
- Ohkubo Y, Kishikawa H, Araki E, et al. Intensive insulin therapy prevents the progression of diabetic microvascular complications in Japanese patients with non-insulin-dependent diabetes mellitus: a randomized prospective 6-year study. Diabetes Res Clin Pract. 1995;28(2):103-117. doi:10.1016/0168-8227(95)01064-k [PubMed: 7587918] [CrossRef]
- 539.
- Intensive blood-glucose control with sulphonylureas or insulin compared with conventional treatment and risk of complications in patients with type 2 diabetes (UKPDS 33). UK Prospective Diabetes Study (UKPDS) Group. Lancet. 1998;352(9131):837-853. doi:10.1016/S0140-6736(98)07019-6 [PubMed: 9742976] [CrossRef]
- 540.
- Effect of intensive blood-glucose control with metformin on complications in overweight patients with type 2 diabetes (UKPDS 34). UK Prospective Diabetes Study (UKPDS) Group. Lancet. 1998;352(9131):854-865. doi:10.1016/S0140-6736(98)07037-8 [PubMed: 9742977] [CrossRef]
- 541.
- Action to Control Cardiovascular Risk in Diabetes Study Group, Gerstein HC, Miller ME, et al. Effects of intensive glucose lowering in type 2 diabetes. N Engl J Med. 2008;358(24):2545-2559. doi:10.1056/NEJMoa0802743 [PMC free article: PMC4551392] [PubMed: 18539917] [CrossRef]
- 542.
- ADVANCE Collaborative Group, Patel A, MacMahon S, et al. Intensive blood glucose control and vascular outcomes in patients with type 2 diabetes. N Engl J Med. 2008;358(24):2560-2572. doi:10.1056/NEJMoa0802987 [PubMed: 18539916] [CrossRef]
- 543.
- de Boer IH, Sun W, Cleary PA, et al. Intensive diabetes therapy and glomerular filtration rate in type 1 diabetes. N Engl J Med. 2011;365(25):2366-2376. doi:10.1056/NEJMoa1111732 [PMC free article: PMC3270008] [PubMed: 22077236] [CrossRef]
- 544.
- Eldor R, Raz I. The individualized target HbA1c: a new method for improving macrovascular risk and glycemia without hypoglycemia and weight gain. Rev Diabet Stud. 2009;6(1):6-12. doi:10.1900/RDS.2009.6.6 [PMC free article: PMC2712916] [PubMed: 19557292] [CrossRef]
- 545.
- Duckworth W, Abraira C, Moritz T, et al. Glucose control and vascular complications in veterans with type 2 diabetes. N Engl J Med. 2009;360(2):129-139. doi:10.1056/NEJMoa0808431 [PubMed: 19092145] [CrossRef]
- 546.
- Gerich JE, Meyer C, Woerle HJ, Stumvoll M. Renal gluconeogenesis: its importance in human glucose homeostasis. Diabetes Care. 2001;24(2):382-391. doi:10.2337/diacare.24.2.382 [PubMed: 11213896] [CrossRef]
- 547.
- Williams ME. Management of diabetes in dialysis patients. Curr Diab Rep. 2009;9(6):466-472. doi:10.1007/s11892-009-0076-8 [PubMed: 19954693] [CrossRef]
- 548.
- Ix JH. Hemoglobin A1c in hemodialysis patients: should one size fit all? Clin J Am Soc Nephrol. 2010;5(9):1539-1541. doi:10.2215/CJN.04410510 [PubMed: 20671219] [CrossRef]
- 549.
- Morgan L, Marenah CB, Jeffcoate WJ, Morgan AG. Glycated proteins as indices of glycaemic control in diabetic patients with chronic renal failure. Diabet Med. 1996;13(6):514-519. doi:10.1002/(SICI)1096-9136(199606)13:6<514::AID-DIA108>3.0.CO;2-4 [PubMed: 8799653] [CrossRef]
- 550.
- Joy MS, Cefalu WT, Hogan SL, Nachman PH. Long-term glycemic control measurements in diabetic patients receiving hemodialysis. Am J Kidney Dis. 2002;39(2):297-307. doi:10.1053/ajkd.2002.30549 [PubMed: 11840370] [CrossRef]
- 551.
- Inaba M, Okuno S, Kumeda Y, et al. Glycated albumin is a better glycemic indicator than glycated hemoglobin values in hemodialysis patients with diabetes: effect of anemia and erythropoietin injection. J Am Soc Nephrol. 2007;18(3):896-903. doi:10.1681/ASN.2006070772 [PubMed: 17267743] [CrossRef]
- 552.
- Riveline JP, Teynie J, Belmouaz S, et al. Glycaemic control in type 2 diabetic patients on chronic haemodialysis: use of a continuous glucose monitoring system. Nephrol Dial Transplant. 2009;24(9):2866-2871. doi:10.1093/ndt/gfp181 [PubMed: 19389864] [CrossRef]
- 553.
- Freedman BI, Shihabi ZK, Andries L, et al. Relationship between assays of glycemia in diabetic subjects with advanced chronic kidney disease. Am J Nephrol. 2010;31(5):375-379. doi:10.1159/000287561 [PubMed: 20299782] [CrossRef]
- 554.
- Ng JM, Cooke M, Bhandari S, Atkin SL, Kilpatrick ES. The effect of iron and erythropoietin treatment on the A1C of patients with diabetes and chronic kidney disease. Diabetes Care. 2010;33(11):2310-2313. doi:10.2337/dc10-0917 [PMC free article: PMC2963485] [PubMed: 20798337] [CrossRef]
- 555.
- Ramirez SP, McCullough KP, Thumma JR, et al. Hemoglobin A(1c) levels and mortality in the diabetic hemodialysis population: findings from the Dialysis Outcomes and Practice Patterns Study (DOPPS). Diabetes Care. 2012;35(12):2527-2532. doi:10.2337/dc12-0573 [PMC free article: PMC3507600] [PubMed: 22912431] [CrossRef]
- 556.
- Shurraw S, Hemmelgarn B, Lin M, et al. Association between glycemic control and adverse outcomes in people with diabetes mellitus and chronic kidney disease: a population-based cohort study. Arch Intern Med. 2011;171(21):1920-1927. doi:10.1001/archinternmed.2011.537 [PubMed: 22123800] [CrossRef]
- 557.
- Sturm G, Lamina C, Zitt E, et al. Association of HbA1c values with mortality and cardiovascular events in diabetic dialysis patients. The INVOR study and review of the literature. PLoS One. 2011;6(5):e20093. doi:10.1371/journal.pone.0020093 [PMC free article: PMC3097236] [PubMed: 21625600] [CrossRef]
- 558.
- Pernicova I, Korbonits M. Metformin—mode of action and clinical implications for diabetes and cancer. Nat Rev Endocrinol. 2014;10(3):143-156. doi:10.1038/nrendo.2013.256 [PubMed: 24393785] [CrossRef]
- 559.
- Amador-Licona N, Guízar-Mendoza J, Vargas E, Sánchez-Camargo G, Zamora-Mata L. The short-term effect of a switch from glibenclamide to metformin on blood pressure and microalbuminuria in patients with type 2 diabetes mellitus. Arch Med Res. 2000;31(6):571-575. doi:10.1016/s0188-4409(00)00241-1 [PubMed: 11257323] [CrossRef]
- 560.
- De Jager J, Kooy A, Lehert P, et al. Effects of short-term treatment with metformin on markers of endothelial function and inflammatory activity in type 2 diabetes mellitus: a randomized, placebo-controlled trial. J Intern Med. 2005;257(1):100-109. doi:10.1111/j.1365-2796.2004.01420.x [PubMed: 15606381] [CrossRef]
- 561.
- Lachin JM, Viberti G, Zinman B, et al. Renal function in type 2 diabetes with rosiglitazone, metformin, and glyburide monotherapy. Clin J Am Soc Nephrol. 2011;6(5):1032-1040. doi:10.2215/cjn.09291010 [PMC free article: PMC3087768] [PubMed: 21454723] [CrossRef]
- 562.
- Kalra S, Das AK, Sahay RK, et al. Consensus recommendations on GLP-1 RA use in the management of type 2 diabetes mellitus: South Asian Task Force. Diabetes Ther. 2019;10(5):1645-1717. doi:10.1007/s13300-019-0669-4 [PMC free article: PMC6778554] [PubMed: 31359367] [CrossRef]
- 563.
- Davies MJ, Bain SC, Atkin SL, et al. Efficacy and safety of liraglutide versus placebo as add-on to glucose-lowering therapy in patients with type 2 diabetes and moderate renal impairment (LIRA-RENAL): a randomized clinical trial. Diabetes Care. 2016;39(2):222-230. doi:10.2337/dc14-2883 [PubMed: 26681713] [CrossRef]
- 564.
- Tuttle KR, Lakshmanan MC, Rayner B, et al. Dulaglutide versus insulin glargine in patients with type 2 diabetes and moderate-to-severe chronic kidney disease (AWARD-7): a multicentre, open-label, randomised trial. Lancet Diabetes Endocrinol. 2018;6(8):605-617. doi:10.1016/S2213-8587(18)30104-9 [PubMed: 29910024] [CrossRef]
- 565.
- Muskiet MHA, Tonneijck L, Huang Y, et al. Lixisenatide and renal outcomes in patients with type 2 diabetes and acute coronary syndrome: an exploratory analysis of the ELIXA randomised, placebo-controlled trial. Lancet Diabetes Endocrinol. 2018;6(11):859-869. doi:10.1016/S2213-8587(18)30268-7 [PubMed: 30292589] [CrossRef]
- 566.
- Bethel MA, Mentz RJ, Merrill P, et al. Microvascular and cardiovascular outcomes according to renal function in patients treated with once-weekly exenatide: insights from the EXSCEL trial. Diabetes Care. 2020;43(2):446-452. doi:10.2337/dc19-1065 [PMC free article: PMC7411285] [PubMed: 31757838] [CrossRef]
- 567.
- Gerstein HC, Colhoun HM, Dagenais GR, et al. Dulaglutide and cardiovascular outcomes in type 2 diabetes (REWIND): a double-blind, randomised placebo-controlled trial. Lancet. 2019;394(10193):121-130. doi:10.1016/s0140-6736(19)31149-3 [PubMed: 31189511] [CrossRef]
- 568.
- Mann JFE, Ørsted DD, Buse JB. Liraglutide and renal outcomes in type 2 diabetes. N Engl J Med. 2017;377(22):2197-2198. doi:10.1056/NEJMc1713042 [PubMed: 29171823] [CrossRef]
- 569.
- Petrie JR, Marso SP, Bain SC, et al. LEADER-4: blood pressure control in patients with type 2 diabetes and high cardiovascular risk: baseline data from the LEADER randomized trial. J Hypertens. 2016;34(6):1140-1150. doi:10.1097/hjh.0000000000000890 [PMC free article: PMC4856174] [PubMed: 26855018] [CrossRef]
- 570.
- Holman RR, Bethel MA, Mentz RJ, et al. Effects of once-weekly exenatide on cardiovascular outcomes in type 2 diabetes. N Engl J Med. 2017;377(13):1228-1239. doi:10.1056/NEJMoa1612917 [PMC free article: PMC9792409] [PubMed: 28910237] [CrossRef]
- 571.
- Kristensen SL, Rørth R, Jhund PS, et al. Cardiovascular, mortality, and kidney outcomes with GLP-1 receptor agonists in patients with type 2 diabetes: a systematic review and meta-analysis of cardiovascular outcome trials. Lancet Diabetes Endocrinol. 2019;7(10):776-785. doi:10.1016/S2213-8587(19)30249-9 [PubMed: 31422062] [CrossRef]
- 572.
- Mentz RJ, Bethel MA, Gustavson S, et al. Baseline characteristics of patients enrolled in the Exenatide Study of Cardiovascular Event Lowering (EXSCEL). Am Heart J. 2017;187:1-9. doi:10.1016/j.ahj.2017.02.005 [PMC free article: PMC9849915] [PubMed: 28454792] [CrossRef]
- 573.
- Perkovic V, Tuttle KR, Rossing P, et al. Effects of semaglutide on chronic kidney disease in patients with type 2 diabetes. N Engl J Med. 2024;391(2):109-121. doi:10.1056/NEJMoa2403347 [PubMed: 38785209] [CrossRef]
- 574.
- Zinman B, Wanner C, Lachin JM, et al. Empagliflozin, cardiovascular outcomes, and mortality in type 2 diabetes. N Engl J Med. 2015;373(22):2117-2128. doi:10.1056/NEJMoa1504720 [PubMed: 26378978] [CrossRef]
- 575.
- Wanner C, Inzucchi SE, Lachin JM, et al. Empagliflozin and progression of kidney disease in type 2 diabetes. N Engl J Med. 2016;375(4):323-334. doi:10.1056/NEJMoa1515920 [PubMed: 27299675] [CrossRef]
- 576.
- Neal B, Perkovic V, Mahaffey KW, et al. Canagliflozin and cardiovascular and renal events in type 2 diabetes. N Engl J Med. 2017;377(7):644-657. doi:10.1056/NEJMoa1611925 [PubMed: 28605608] [CrossRef]
- 577.
- Wiviott SD, Raz I, Bonaca MP, et al. Dapagliflozin and cardiovascular outcomes in type 2 diabetes. N Engl J Med. 2019;380(4):347-357. doi:10.1056/NEJMoa1812389 [PubMed: 30415602] [CrossRef]
- 578.
- Perkovic V, Jardine MJ, Neal B, et al. Canagliflozin and renal outcomes in type 2 diabetes and nephropathy. N Engl J Med. 2019;380(24):2295-2306. doi:10.1056/NEJMoa1811744 [PubMed: 30990260] [CrossRef]
- 579.
- Heerspink HJL, Stefánsson BV, Correa-Rotter R, et al. Dapagliflozin in patients with chronic kidney disease. N Engl J Med. 2020;383(15):1436-1446. doi:10.1056/NEJMoa2024816 [PubMed: 32970396] [CrossRef]
- 580.
- Wheeler DC, Stefansson BV, Batiushin M, et al. The dapagliflozin and prevention of adverse outcomes in chronic kidney disease (DAPA-CKD) trial: baseline characteristics. Nephrol Dial Transplant. 2020;35(10):1700-1711. doi:10.1093/ndt/gfaa234 [PMC free article: PMC7538235] [PubMed: 32862232] [CrossRef]
- 581.
- Cannon CP, McGuire DK, Pratley R, et al. Design and baseline characteristics of the eValuation of ERTugliflozin effIcacy and Safety CardioVascular outcomes trial (VERTIS-CV). Am Heart J. 2018;206:11-23. doi:10.1016/j.ahj.2018.08.016 [PubMed: 30290289] [CrossRef]
- 582.
- Cannon CP, Pratley R, Dagogo-Jack S, et al. Cardiovascular outcomes with ertugliflozin in type 2 diabetes. N Engl J Med. 2020;383(15):1425-1435. doi:10.1056/NEJMoa2004967 [PubMed: 32966714] [CrossRef]
- 583.
- McMurray JJV, Solomon SD, Inzucchi SE, et al. Dapagliflozin in patients with heart failure and reduced ejection fraction. N Engl J Med. 2019;381(21):1995-2008. doi:10.1056/NEJMoa1911303 [PubMed: 31535829] [CrossRef]
- 584.
- Petrie MC, Verma S, Docherty KF, et al. Effect of dapagliflozin on worsening heart failure and cardiovascular death in patients with heart failure with and without diabetes. JAMA. 2020;323(14):1353-1368. doi:10.1001/jama.2020.1906 [PMC free article: PMC7157181] [PubMed: 32219386] [CrossRef]
- 585.
- Packer M, Anker SD, Butler J, et al. Cardiovascular and renal outcomes with empagliflozin in heart failure. N Engl J Med. 2020;383(15):1413-1424. doi:10.1056/NEJMoa2022190 [PubMed: 32865377] [CrossRef]
- 586.
- Anker SD, Butler J, Filippatos G, et al. Effect of empagliflozin on cardiovascular and renal outcomes in patients with heart failure by baseline diabetes status: results from the EMPEROR-Reduced trial. Circulation. 2021;143(4):337-349. doi:10.1161/circulationaha.120.051824 [PMC free article: PMC7834911] [PubMed: 33175585] [CrossRef]
- 587.
- Anker SD, Butler J, Filippatos G, et al. Empagliflozin in heart failure with a preserved ejection fraction. N Engl J Med. 2021;385(16):1451-1461. doi:10.1056/NEJMoa2107038 [PubMed: 34449189] [CrossRef]
- 588.
- Anker SD, Butler J, Filippatos G, et al. Baseline characteristics of patients with heart failure with preserved ejection fraction in the EMPEROR-Preserved trial. Eur J Heart Fail. 2020;22(12):2383-2392. doi:10.1002/ejhf.2064 [PubMed: 33251670] [CrossRef]
- 589.
- Timmons JG, Littlejohn L, Boyle JG, Petrie JR. Recent developments in adjunct therapies for type 1 diabetes. Expert Opin Investig Drugs. 2022;31(12):1311-1320. doi:10.1080/13543784.2022.2159806 [PubMed: 36655950] [CrossRef]
- 590.
- Nagase M, Fujita T. Mineralocorticoid receptor activation in obesity hypertension. Hypertens Res. 2009;32(8):649-657. doi:10.1038/hr.2009.86 [PubMed: 19521418] [CrossRef]
- 591.
- Barrera-Chimal J, Lima-Posada I, Bakris GL, Jaisser F. Mineralocorticoid receptor antagonists in diabetic kidney disease—mechanistic and therapeutic effects. Nat Rev Nephrol. 2022;18(1):56-70. doi:10.1038/s41581-021-00490-8 [PubMed: 34675379] [CrossRef]
- 592.
- Verma S, Vaidya A, Subudhi S, Waikar SS. Aldosterone in chronic kidney disease and renal outcomes. Eur Heart J. 2022;43(38):3781-3791. doi:10.1093/eurheartj/ehac352 [PMC free article: PMC10147385] [PubMed: 36219773] [CrossRef]
- 593.
- Bakris GL, Agarwal R, Anker SD, et al. Effect of finerenone on chronic kidney disease outcomes in type 2 diabetes. N Engl J Med. 2020;383(23):2219-2229. doi:10.1056/NEJMoa2025845 [PubMed: 33264825] [CrossRef]
- 594.
- Pitt B, Filippatos G, Agarwal R, et al. Cardiovascular events with finerenone in kidney disease and type 2 diabetes. N Engl J Med. 2021;385(24):2252-2263. doi:10.1056/NEJMoa2110956 [PubMed: 34449181] [CrossRef]
- 595.
- Heerspink HJL, Birkenfeld AL, Cherney DZI, et al. Rationale and design of a randomised phase III registration trial investigating finerenone in participants with type 1 diabetes and chronic kidney disease: the FINE-ONE trial. Diabetes Res Clin Pract. 2023;204:110908. doi:10.1016/j.diabres.2023.110908 [PubMed: 37805000] [CrossRef]
- 596.
- Heerspink HJL, Parving HH, Andress DL, et al. Atrasentan and renal events in patients with type 2 diabetes and chronic kidney disease (SONAR): a double-blind, randomised, placebo-controlled trial. Lancet. 2019;393(10184):1937-1947. doi:10.1016/s0140-6736(19)30772-x [PubMed: 30995972] [CrossRef]
- 597.
- Mann JF, Green D, Jamerson K, et al. Avosentan for overt diabetic nephropathy. J Am Soc Nephrol. 2011;21(3):527-535. doi:10.1681/ASN.2009060593 [PMC free article: PMC2831858] [PubMed: 20167702] [CrossRef]
- 598.
- Hoekman J, Lambers Heerspink HJ, Viberti G, Green D, Mann JF, de Zeeuw D. Predictors of congestive heart failure after treatment with an endothelin receptor antagonist. Clin J Am Soc Nephrol. 2013;9(3):490-498. doi:10.2215/CJN.07040713 [PMC free article: PMC3944766] [PubMed: 24408122] [CrossRef]
- 599.
- Balamuthusamy S, Srinivasan L, Verma M, et al. Renin angiotensin system blockade and cardiovascular outcomes in patients with chronic kidney disease and proteinuria: a metaanalysis. Am Heart J. 2008;155(5):791-805. doi:10.1016/j.ahj.2008.01.031 [PubMed: 18440325] [CrossRef]
- 600.
- ACE Inhibitors in Diabetic Nephropathy Trialist Group. Should all patients with type 1 diabetes mellitus and microalbuminuria receive angiotensin-converting enzyme inhibitors? A meta-analysis of individual patient data. Ann Intern Med. 2001;134(5):370-379. doi:10.7326/0003-4819-134-5-200103060-00009 [PubMed: 11242497] [CrossRef]
- 601.
- Lemley KV. When to initiate ACEI/ARB therapy in patients with type 1 and 2 diabetes. Pediatr Nephrol. 2010;25(10):2021-2034. doi:10.1007/s00467-010-1498-x [PubMed: 20352458] [CrossRef]
- 602.
- Lewis EJ, Hunsicker LG, Bain RP, Rohde RD. The effect of angiotensin-converting-enzyme inhibition on diabetic nephropathy. The Collaborative Study Group. N Engl J Med. 1993;329(20):1456-1462. doi:10.1056/NEJM199311113292004 [PubMed: 8413456] [CrossRef]
- 603.
- Mauer M, Zinman B, Gardiner R, et al. Renal and retinal effects of enalapril and losartan in type 1 diabetes. N Engl J Med. 2009;361(1):40-51. doi:10.1056/NEJMoa0808400 [PMC free article: PMC2978030] [PubMed: 19571282] [CrossRef]
- 604.
- Chaturvedi N, The EUCLID Study Group. Randomised placebo-controlled trial of lisinopril in normotensive patients with insulin-dependent diabetes and normoalbuminuria or microalbuminuria. Lancet. 1997;349(9068):1787-1792. doi:10.1016/S0140-6736(96)10244-0 [PubMed: 9269212] [CrossRef]
- 605.
- Effects of ramipril on cardiovascular and microvascular outcomes in people with diabetes mellitus: results of the HOPE study and MICRO-HOPE substudy. Heart Outcomes Prevention Evaluation Study Investigators. Lancet. 2000;355(9200):253-259. doi:10.1016/S0140-6736(99)12323-7 [PubMed: 10675071] [CrossRef]
- 606.
- Ruggenenti P, Fassi A, Ilieva AP, et al. Preventing microalbuminuria in type 2 diabetes. N Engl J Med. 2004;351(19):1941-1951. doi:10.1056/NEJMoa042167 [PubMed: 15516697] [CrossRef]
- 607.
- Ravid M, Brosh D, Levi Z, Bar-Dayan Y, Ravid D, Rachmani R. Use of enalapril to attenuate decline in renal function in normotensive, normoalbuminuric patients with type 2 diabetes mellitus. A randomized, controlled trial. Ann Intern Med 1998;128(12 Pt 1):982-988. doi:10.7326/0003-4819-128-12_part_1-199806150-00004 [PubMed: 9625684] [CrossRef]
- 608.
- Bilous R, Chaturvedi N, Sjølie AK, et al. Effect of candesartan on microalbuminuria and albumin excretion rate in diabetes: three randomized trials. Ann Intern Med. 2009;151(1):11-20. doi:10.7326/0003-4819-151-1-200907070-00120 [PubMed: 19451554] [CrossRef]
- 609.
- Mann JF, Schmieder RE, Dyal L, et al. Effect of telmisartan on renal outcomes: a randomized trial. Ann Intern Med. 2009;151(1):1-10. doi:10.7326/0003-4819-151-1-200907070-00122 [PubMed: 19451556] [CrossRef]
- 610.
- Haller H, Ito S, Izzo JL, Jr., et al. Olmesartan for the delay or prevention of microalbuminuria in type 2 diabetes. N Engl J Med. 2011;364(10):907-917. doi:10.1056/NEJMoa1007994 [PubMed: 21388309] [CrossRef]
- 611.
- Parving HH, Lehnert H, Bröchner-Mortensen J, et al. The effect of irbesartan on the development of diabetic nephropathy in patients with type 2 diabetes. N Engl J Med. 2001;345(12):870-878. doi:10.1056/NEJMoa011489 [PubMed: 11565519] [CrossRef]
- 612.
- Brenner BM, Cooper ME, de Zeeuw D, et al. Effects of losartan on renal and cardiovascular outcomes in patients with type 2 diabetes and nephropathy. N Engl J Med. 2001;345(12):861-869. doi:10.1056/NEJMoa011161 [PubMed: 11565518] [CrossRef]
- 613.
- Lewis EJ, Hunsicker LG, Clarke WR, et al. Renoprotective effect of the angiotensin-receptor antagonist irbesartan in patients with nephropathy due to type 2 diabetes. N Engl J Med. 2001;345(12):851-860. doi:10.1056/NEJMoa011303 [PubMed: 11565517] [CrossRef]
- 614.
- ONTARGET Investigators, Yusuf S, Teo KK, et al. Telmisartan, ramipril, or both in patients at high risk for vascular events. N Engl J Med. 2008;358(15):1547-1559. doi:10.1056/NEJMoa0801317 [PubMed: 18378520] [CrossRef]
- 615.
- Barnett AH, Bain SC, Bouter P, et al. Angiotensin-receptor blockade versus converting-enzyme inhibition in type 2 diabetes and nephropathy. N Engl J Med. 2004;351(19):1952-1961. doi:10.1056/NEJMoa042274 [PubMed: 15516696] [CrossRef]
- 616.
- Vejakama P, Thakkinstian A, Lertrattananon D, Ingsathit A, Ngarmukos C, Attia J. Reno-protective effects of renin-angiotensin system blockade in type 2 diabetic patients: a systematic review and network meta-analysis. Diabetologia. 2012;55(3):566-578. doi:10.1007/s00125-011-2398-8 [PMC free article: PMC3268972] [PubMed: 22189484] [CrossRef]
- 617.
- Lv J, Perkovic V, Foote CV, Craig ME, Craig JC, Strippoli GF. Antihypertensive agents for preventing diabetic kidney disease. Cochrane Database Syst Rev. 2012;12:CD004136. doi:10.1002/14651858.CD004136.pub3 [PMC free article: PMC11357690] [PubMed: 23235603] [CrossRef]
- 618.
- Strippoli GF, Craig M, Deeks JJ, Schena FP, Craig JC. Effects of angiotensin converting enzyme inhibitors and angiotensin II receptor antagonists on mortality and renal outcomes in diabetic nephropathy: systematic review. BMJ. 2004;329(7470):828. doi:10.1136/bmj.38237.585000.7C [PMC free article: PMC521570] [PubMed: 15459003] [CrossRef]
- 619.
- Mann JFE, Anderson C, Gao P, et al. Dual inhibition of the renin-angiotensin system in high-risk diabetes and risk for stroke and other outcomes: results of the ONTARGET trial. J Hypertens. 2013;31(2):414-421. doi:10.1097/HJH.0b013e32835bf7b0 [PubMed: 23249829] [CrossRef]
- 620.
- Croom KF, Curran MP, Goa KL, Perry CM. Irbesartan: a review of its use in hypertension and in the management of diabetic nephropathy. Drugs. 2004;64(9):999-1028. doi:10.2165/00003495-200464090-00011 [PubMed: 15101793] [CrossRef]
- 621.
- Mogensen CE, Neldam S, Tikkanen I, et al. Randomised controlled trial of dual blockade of renin-angiotensin system in patients with hypertension, microalbuminuria, and non-insulin dependent diabetes: the candesartan and lisinopril microalbuminuria (CALM) study. BMJ. 2000;321(7274):1440-1444. doi:10.1136/bmj.321.7274.1440 [PMC free article: PMC27545] [PubMed: 11110735] [CrossRef]
- 622.
- Bakris GL, Ruilope L, Locatelli F, et al. Treatment of microalbuminuria in hypertensive subjects with elevated cardiovascular risk: results of the IMPROVE trial. Kidney Int. 2007;72(7):879-885. doi:10.1038/sj.ki.5002455 [PubMed: 17667984] [CrossRef]
- 623.
- Fried LF, Emanuele N, Zhang JH, et al. Combined angiotensin inhibition for the treatment of diabetic nephropathy. N Engl J Med. 2013;369(20):1892-1903. doi:10.1056/NEJMoa1303154 [PubMed: 24206457] [CrossRef]
- 624.
- Parving HH, Persson F, Lewis JB, Lewis EJ, Hollenberg NK, AVOID Study Investigators. Aliskiren combined with losartan in type 2 diabetes and nephropathy. N Engl J Med. 2008;358(23):2433-2446. doi:10.1056/NEJMoa0708379 [PubMed: 18525041] [CrossRef]
- 625.
- Parving HH, Brenner BM, McMurray JJ, et al. Cardiorenal end points in a trial of aliskiren for type 2 diabetes. N Engl J Med. 2012;367(23):2204-2213. doi:10.1056/NEJMoa1208799 [PubMed: 23121378] [CrossRef]
- 626.
- Bangalore S, Kumar S, Lobach I, Messerli FH. Blood pressure targets in subjects with type 2 diabetes mellitus/impaired fasting glucose: observations from traditional and bayesian random-effects meta-analyses of randomized trials. Circulation. 2011;123(24):2799-2810. doi:10.1161/CIRCULATIONAHA.110.016337 [PubMed: 21632497] [CrossRef]
- 627.
- Klahr S, Levey AS, Beck GJ, et al. The effects of dietary protein restriction and blood-pressure control on the progression of chronic renal disease. Modification of Diet in Renal Disease Study Group. N Engl J Med. 1994;330(13):877-884. doi:10.1056/NEJM199403313301301 [PubMed: 8114857] [CrossRef]
- 628.
- Walker WG, Hermann J, Anderson J. Racial differences in renal protective effect of enalapril vs hydrochlorothiazide in randomized doubly blinded trial in hypertensive NIDDM (Abstract). J Am Soc Nephrol. 1993;4:A310
- 629.
- McClellan WM, Warnock DG, Judd S, et al. Albuminuria and racial disparities in the risk for ESRD. J Am Soc Nephrol. 2011;22(9):1721-1728. doi:10.1681/ASN.2010101085 [PMC free article: PMC3171942] [PubMed: 21868498] [CrossRef]
- 630.
- Murea M, Freedman BI. Essential hypertension and risk of nephropathy: a reappraisal. Curr Opin Nephrol Hypertens. 2010;19(3):235-241. doi:10.1097/MNH.0b013e3283366344 [PMC free article: PMC2904692] [PubMed: 20051853] [CrossRef]
- 631.
- Weir MR, Bakris GL, Weber MA, et al. Renal outcomes in hypertensive Black patients at high cardiovascular risk. Kidney Int. 2012;81(6):568-576. doi:10.1038/ki.2011.417 [PubMed: 22189843] [CrossRef]
- 632.
- James PA, Oparil S, Carter BL, et al. 2014 evidence-based guideline for the management of high blood pressure in adults: report from the panel members appointed to the Eighth Joint National Committee (JNC 8). JAMA. 2014;311(5):507-520. doi:10.1001/jama.2013.284427 [PubMed: 24352797] [CrossRef]
- 633.
- Jain R, Stone JA, Agarwal G, et al. Canadian Cardiovascular Harmonized National Guideline Endeavour (C-CHANGE) guideline for the prevention and management of cardiovascular disease in primary care: 2022 update. CMAJ. 2022;194(43):E1460-E1480. doi:10.1503/cmaj.220138 [PMC free article: PMC9828999] [PubMed: 36343954] [CrossRef]
- 634.
- Williams B, Mancia G, Spiering W, et al. 2018 Practice guidelines for the management of arterial hypertension of the European Society of Hypertension and the European Society of Cardiology: ESH/ESC Task Force for the Management of Arterial Hypertension. J Hypertens. 2018;36(12):2284-2309. doi:10.1097/hjh.0000000000001961 [PubMed: 30379783] [CrossRef]
- 635.
- Kidney Disease: Improving Global Outcomes (KDIGO) Blood Pressure Work Group. KDIGO 2021 clinical practice guideline for the management of blood pressure in chronic kidney disease. Kidney Int. 2021;99(3s):S1-S87. doi:10.1016/j.kint.2020.11.003 [PubMed: 33637192] [CrossRef]
- 636.
- Barrett-Connor E, Grundy SM, Holdbrook MJ. Plasma lipids and diabetes mellitus in an adult community. Am J Epidemiol. 1982;115(5):657-663. doi:10.1093/oxfordjournals.aje.a113348 [PubMed: 7081197] [CrossRef]
- 637.
- Feingold KR, Grunfeld C, Pang M, Doerrler W, Krauss RM. LDL subclass phenotypes and triglyceride metabolism in non-insulin-dependent diabetes. Arterioscler Thromb. 1992;12(12):1496-1502. doi:10.1161/01.atv.12.12.1496 [PubMed: 1450181] [CrossRef]
- 638.
- Quaschning T, Schömig M, Keller M, et al. Non-insulin-dependent diabetes mellitus and hypertriglyceridemia impair lipoprotein metabolism in chronic hemodialysis patients. J Am Soc Nephrol. 1999;10(2):332-341. doi:10.1681/ASN.V102332 [PubMed: 10215333] [CrossRef]
- 639.
- Chonchol M, Cook T, Kjekshus J, Pedersen TR, Lindenfeld J. Simvastatin for secondary prevention of all-cause mortality and major coronary events in patients with mild chronic renal insufficiency. Am J Kidney Dis. 2007;49(3):373-382. doi:10.1053/j.ajkd.2006.11.043 [PubMed: 17336698] [CrossRef]
- 640.
- Colhoun HM, Betteridge DJ, Durrington PN, et al. Effects of atorvastatin on kidney outcomes and cardiovascular disease in patients with diabetes: an analysis from the Collaborative Atorvastatin Diabetes Study (CARDS). Am J Kidney Dis. 2009;54(5):810-819. doi:10.1053/j.ajkd.2009.03.022 [PubMed: 19540640] [CrossRef]
- 641.
- Collins R, Armitage J, Parish S, Sleigh P, Peto R, Heart Protection Study Collaborative Group. MRC/BHF Heart Protection Study of cholesterol-lowering with simvastatin in 5963 people with diabetes: a randomised placebo-controlled trial. Lancet. 2003;361(9374):2005-2016. doi:10.1016/s0140-6736(03)13636-7 [PubMed: 12814710] [CrossRef]
- 642.
- Tonelli M, Keech A, Shepherd J, et al. Effect of pravastatin in people with diabetes and chronic kidney disease. J Am Soc Nephrol. 2005;16(12):3748-3754. doi:10.1681/ASN.2005070779 [PubMed: 16251235] [CrossRef]
- 643.
- Baigent C, Landray MJ, Reith C, et al. The effects of lowering LDL cholesterol with simvastatin plus ezetimibe in patients with chronic kidney disease (Study of Heart and Renal Protection): a randomised placebo-controlled trial. Lancet. 2011;377(9784):2181-2192. doi:10.1016/s0140-6736(11)60739-3 [PMC free article: PMC3145073] [PubMed: 21663949] [CrossRef]
- 644.
- Wanner C, Krane V, März W, et al. Atorvastatin in patients with type 2 diabetes mellitus undergoing hemodialysis. N Engl J Med. 2005;353(3):238-248. doi:10.1056/NEJMoa043545 [PubMed: 16034009] [CrossRef]
- 645.
- Fellström BC, Jardine AG, Schmieder RE, et al. Rosuvastatin and cardiovascular events in patients undergoing hemodialysis. N Engl J Med. 2009;360(14):1395-1407. doi:10.1056/NEJMoa0810177 [PubMed: 19332456] [CrossRef]
- 646.
- Holdaas H, Holme I, Schmieder RE, et al. Rosuvastatin in diabetic hemodialysis patients. J Am Soc Nephrol. 2011;22(7):1335-1341. doi:10.1681/ASN.2010090987 [PMC free article: PMC3137581] [PubMed: 21566054] [CrossRef]
- 647.
- Jardine AG, Holdaas H, Fellström B, et al. Fluvastatin prevents cardiac death and myocardial infarction in renal transplant recipients: post-hoc subgroup analyses of the ALERT Study. Am J Transplant. 2004;4(6):988-995. doi:10.1111/j.1600-6143.2004.00445.x [PubMed: 15147434] [CrossRef]
- 648.
- Tonelli M, Collins D, Robins S, Bloomfield H, Curhan GC, Veterans’ Affairs High-Density Lipoprotein Intervention Trial (VA-HIT) Investigators. Gemfibrozil for secondary prevention of cardiovascular events in mild to moderate chronic renal insufficiency. Kidney Int. 2004;66(3):1123-1130. doi:10.1111/j.1523-1755.2004.00862.x [PubMed: 15327407] [CrossRef]
- 649.
- Wen SF, Huang TP, Moorthy AV. Effects of low-protein diet on experimental diabetic nephropathy in the rat. J Lab Clin Med. 1985;106(5):589-597. [PubMed: 3903011]
- 650.
- Sällström J, Carlström M, Olerud J, et al. High-protein-induced glomerular hyperfiltration is independent of the tubuloglomerular feedback mechanism and nitric oxide synthases. Am J Physiol Regul Integr Comp Physiol. 2010;299(5):R1263-R1268. doi:10.1152/ajpregu.00649.2009 [PubMed: 20739607] [CrossRef]
- 651.
- Wiseman MJ, Bognetti E, Dodds R, Keen H, Viberti GC. Changes in renal function in response to protein restricted diet in type 1 (insulin-dependent) diabetic patients. Diabetologia. 1987;30(3):154-159. doi:10.1007/BF00274220 [PubMed: 3582821] [CrossRef]
- 652.
- Cohen D, Dodds R, Viberti G. Effect of protein restriction in insulin dependent diabetics at risk of nephropathy. Br Med J (Clin Res Ed). 1987;294(6575):795-798. doi:10.1136/bmj.294.6575.795 [PMC free article: PMC1245861] [PubMed: 3105747] [CrossRef]
- 653.
- Pedersen MM, Mogensen CE, Jørgensen FS, Møller B, Lykke G, Pedersen O. Renal effects from limitation of high dietary protein in normoalbuminuric diabetic patients. Kidney Int Suppl. 1989;27:S115-S121. [PubMed: 2636645]
- 654.
- Dullaart RP, Beusekamp B, Meijer S, van Doormaal JJ, Sluiter WJ. Long-term effects of protein-restricted diet on albuminuria and renal function in IDDM patients without clinical nephropathy and hypertension. Diabetes Care. 1993;16(2):483-492. doi:10.2337/diacare.16.2.483 [PubMed: 8432221] [CrossRef]
- 655.
- Tuttle KR, Puhlman ME, Cooney SK, Short RA. Effects of amino acids and glucagon on renal hemodynamics in type 1 diabetes. Am J Physiol Renal Physiol. 2002;282(1):F103-F112. doi:10.1152/ajprenal.00155.2001 [PubMed: 11739118] [CrossRef]
- 656.
- Ciavarella A, Di Mizio G, Stefoni S, Borgnino LC, Vannini P. Reduced albuminuria after dietary protein restriction in insulin-dependent diabetic patients with clinical nephropathy. Diabetes Care. 1987;10(4):407-413. doi:10.2337/diacare.10.4.407 [PubMed: 3622197] [CrossRef]
- 657.
- Evanoff G, Thompson C, Brown J, Weinman E. Prolonged dietary protein restriction in diabetic nephropathy. Arch Intern Med. 1989;149(5):1129-1133. doi:10.1001/archinte.1989.00390050099020 [PubMed: 2719506] [CrossRef]
- 658.
- Walker JD, Bending JJ, Dodds RA, et al. Restriction of dietary protein and progression of renal failure in diabetic nephropathy. Lancet. 1989;2(8677):1411-1415. doi:10.1016/s0140-6736(89)92032-1 [PubMed: 2574360] [CrossRef]
- 659.
- Zeller K, Whittaker E, Sullivan L, Raskin P, Jacobson HR. Effect of restricting dietary protein on the progression of renal failure in patients with insulin-dependent diabetes mellitus. N Engl J Med. 1991;324(2):78-84. doi:10.1056/NEJM199101103240202 [PubMed: 1984187] [CrossRef]
- 660.
- Hansen HP, Tauber-Lassen E, Jensen BR, Parving HH. Effect of dietary protein restriction on prognosis in patients with diabetic nephropathy. Kidney Int. 2002;62(1):220-228. doi:10.1046/j.1523-1755.2002.00421.x [PubMed: 12081581] [CrossRef]
- 661.
- Pijls LT, de Vries H, van Eijk JT, Donker AJ. Protein restriction, glomerular filtration rate and albuminuria in patients with type 2 diabetes mellitus: a randomized trial. Eur J Clin Nutr. 2002;56(12):1200-1207. doi:10.1038/sj.ejcn.1601474 [PubMed: 12494305] [CrossRef]
- 662.
- Pan Y, Guo LL, Jin HM. Low-protein diet for diabetic nephropathy: a meta-analysis of randomized controlled trials. Am J Clin Nutr. 2008;88(3):660-666. doi:10.1093/ajcn/88.3.660 [PubMed: 18779281] [CrossRef]
- 663.
- Meloni C, Tatangelo P, Cipriani S, et al. Adequate protein dietary restriction in diabetic and nondiabetic patients with chronic renal failure. J Ren Nutr. 2004;14(4):208-213. doi:10.1053/j.jrn.2004.07.011 [PubMed: 15483780] [CrossRef]
- 664.
- McGuire S. Institute of Medicine. 2010. Strategies to Reduce Sodium Intake in the United States. Washington, DC: The National Academies Press. Adv Nutr. 2010;1(1):49-50. doi:10.3945/an.110.1002 [PMC free article: PMC3042781] [PubMed: 22043452] [CrossRef]
- 665.
- McGuire S. U.S. Department of Agriculture and U.S. Department of Health and Human Services, Dietary Guidelines for Americans, 2010. 7th Edition, Washington, DC: U.S. Government Printing Office, January 2011. Adv Nutr. 2011;2(3):293-294. doi:10.3945/an.111.00043010.3945/an.111.000430 [PMC free article: PMC3090168] [PubMed: 22332062] [CrossRef] [CrossRef]
- 666.
- Centers for Disease Control and Prevention (CDC). Usual sodium intakes compared with current dietary guidelines—United States, 2005–2008. MMWR Morb Mortal Wkly Rep. 2011;60(41):1413-1417. [PubMed: 22012113]
- 667.
- Suckling RJ, He FJ, Macgregor GA. Altered dietary salt intake for preventing and treating diabetic kidney disease. Cochrane Database Syst Rev. 2010;(12):CD006763. doi:10.1002/14651858.CD006763.pub2 [PubMed: 21154374] [CrossRef]
- 668.
- Sacks FM, Svetkey LP, Vollmer WM, et al. Effects on blood pressure of reduced dietary sodium and the Dietary Approaches to Stop Hypertension (DASH) diet. DASH-Sodium Collaborative Research Group. N Engl J Med. 2001;344(1):3-10. doi:10.1056/NEJM200101043440101 [PubMed: 11136953] [CrossRef]
- 669.
- Lin J, Fung TT, Hu FB, Curhan GC. Association of dietary patterns with albuminuria and kidney function decline in older white women: a subgroup analysis from the Nurses’ Health Study. Am J Kidney Dis. 2011;57(2):245-254. doi:10.1053/j.ajkd.2010.09.027 [PMC free article: PMC3026604] [PubMed: 21251540] [CrossRef]
- 670.
- Perkins BA, Rabbani N, Weston A, et al. Serum levels of advanced glycation endproducts and other markers of protein damage in early diabetic nephropathy in type 1 diabetes. PLoS One. 2012;7(4):e35655. doi:10.1371/journal.pone.0035655 [PMC free article: PMC3338454] [PubMed: 22558190] [CrossRef]
- 671.
- RamachandraRao SP, Zhu Y, Ravasi T, et al. Pirfenidone is renoprotective in diabetic kidney disease. J Am Soc Nephrol. 2009;20(8):1765-1775. doi:10.1681/ASN.2008090931 [PMC free article: PMC2723978] [PubMed: 19578007] [CrossRef]
- 672.
- Shimizu T, Fukagawa M, Kuroda T, et al. Pirfenidone prevents collagen accumulation in the remnant kidney in rats with partial nephrectomy. Kidney Int Suppl. 1997;63:S239-S243. [PubMed: 9407470]
- 673.
- Cho ME, Smith DC, Branton MH, Penzak SR, Kopp JB. Pirfenidone slows renal function decline in patients with focal segmental glomerulosclerosis. Clin J Am Soc Nephrol. 2007;2(5):906-913. doi:10.2215/CJN.01050207 [PubMed: 17702727] [CrossRef]
- 674.
- Sharma K, Ix JH, Mathew AV, et al. Pirfenidone for diabetic nephropathy. J Am Soc Nephrol. 2011;22(6):1144-1151. doi:10.1681/ASN.2010101049 [PMC free article: PMC3103734] [PubMed: 21511828] [CrossRef]
- 675.
- Hagiwara S, Kantharidis P, Cooper ME. What are new avenues for renal protection, in addition to RAAS inhibition? Curr Hypertens Rep. 2012;14(2):100-110. doi:10.1007/s11906-012-0251-1 [PubMed: 22298106] [CrossRef]
- 676.
- Zieman SJ, Melenovsky V, Clattenburg L, et al. Advanced glycation endproduct crosslink breaker (alagebrium) improves endothelial function in patients with isolated systolic hypertension. J Hypertens. 2007;25(3):577-583. doi:10.1097/HJH.0b013e328013e7dd [PubMed: 17278974] [CrossRef]
- 677.
- Kass DA, Shapiro EP, Kawaguchi M, et al. Improved arterial compliance by a novel advanced glycation end-product crosslink breaker. Circulation. 2001;104(13):1464-1470. doi:10.1161/hc3801.097806 [PubMed: 11571237] [CrossRef]
- 678.
- Shan D, Wu HM, Yuan QY, Li J, Zhou RL, Liu GJ. Pentoxifylline for diabetic kidney disease. Cochrane Database Syst Rev. 2012;(2):CD006800. doi:10.1002/14651858.CD006800.pub2 [PubMed: 22336824] [CrossRef]
- 679.
- Goicoechea M, García de Vinuesa S, Quiroga B, et al. Effects of pentoxifylline on inflammatory parameters in chronic kidney disease patients: a randomized trial. J Nephrol. 2012;25(6):969-975. doi:10.5301/jn.5000077 [PubMed: 22241639] [CrossRef]
- 680.
- Komers R. Rho kinase inhibition in diabetic kidney disease. Curr Opin Nephrol Hypertens. 2011;20(1):77-83. doi:10.1097/MNH.0b013e32834131f8 [PubMed: 21076299] [CrossRef]
- 681.
- Gaede P, Lund-Andersen H, Parving HH, Pedersen O. Effect of a multifactorial intervention on mortality in type 2 diabetes. N Engl J Med. 2008;358(6):580-591. doi:10.1056/NEJMoa0706245 [PubMed: 18256393] [CrossRef]
- 682.
- TODAY Study Group. Rapid rise in hypertension and nephropathy in youth with type 2 diabetes: the TODAY clinical trial. Diabetes Care. 2013;36(6):1735-1741. doi:10.2337/dc12-2420 [PMC free article: PMC3661847] [PubMed: 23704672] [CrossRef]
- 683.
- Berkowitz RI, Marcus MD, Anderson BJ, et al. Adherence to a lifestyle program for youth with type 2 diabetes and its association with treatment outcome in the TODAY clinical trial. Pediatr Diabetes. 2018;19(2):191-198. doi:10.1111/pedi.12555 [PMC free article: PMC5748014] [PubMed: 28664624] [CrossRef]
- 684.
- Trief PM, Kalichman SC, Wang D, et al. Medication adherence in young adults with youth-onset type 2 diabetes: iCount, an observational study. Diabetes Res Clin Pract. 2022;184:109216. doi:10.1016/j.diabres.2022.109216 [PubMed: 35085644] [CrossRef]
- 685.
- Copeland KC, Zeitler P, Geffner M, et al. Characteristics of adolescents and youth with recent-onset type 2 diabetes: the TODAY cohort at baseline. J Clin Endocrinol Metab. 2011;96(1):159-167. doi:10.1210/jc.2010-1642 [PMC free article: PMC3038479] [PubMed: 20962021] [CrossRef]
- 686.
- Laffel LMB, Tamborlane WV, Yver A, et al. Pharmacokinetic and pharmacodynamic profile of the sodium-glucose co-transporter-2 inhibitor empagliflozin in young people with type 2 diabetes: a randomized trial. Diabet Med. 2018;35(8):1096-1104. doi:10.1111/dme.13629 [PMC free article: PMC6099360] [PubMed: 29655290] [CrossRef]
- 687.
- Bjornstad P, Laffel L, Tamborlane WV, et al. Acute effect of empagliflozin on fractional excretion of sodium and eGFR in youth with type 2 diabetes. Diabetes Care. 2018;41(8):e129-e130. doi:10.2337/dc18-0394 [PMC free article: PMC6054503] [PubMed: 29941496] [CrossRef]
- 688.
- Sharkey TP, Root HF. Infection of the urinary tract in diabetes. JAMA. 1935;104(25):2231-2235. doi:10.1001/jama.1935.02760250009003 [CrossRef]
- 689.
- Baldwin AD, Root HF. Infections of the upper urinary tract in the diabetic patient. N Engl J Med. 1940;223(7):244-250. doi:10.1056/NEJM194008152230703 [CrossRef]
- 690.
- Robbins SL, Tucker AW, Jr. The cause of death in diabetes. A report of 307 autopsied cases. N Engl J Med 1944;231(26):865-868. doi:10.1056/NEJM194412282312601 [CrossRef]
- 691.
- Edmondson HA, Martin HE, Evans N. Necrosis of renal papillae and acute pyelonephritis in diabetes mellitus. Arch Intern Med (Chic). 1947;79(2):148-175. doi:10.1001/archinte.1947.00220080036002 [PubMed: 20285219] [CrossRef]
- 692.
- Aye RC. Renal papillary necrosis. Diabetes. 1954;3(2):124-128. doi:10.2337/diab.3.2.124 [PubMed: 13161733] [CrossRef]
- 693.
- Zhanel GG, Harding GK, Nicolle LE. Asymptomatic bacteriuria in patients with diabetes mellitus. Rev Infect Dis. 1991;13(1):150-154. doi:10.1093/clinids/12.5.150 [PubMed: 2017615] [CrossRef]
- 694.
- Renko M, Tapanainen P, Tossavainen P, Pokka T, Uhari M. Meta-analysis of the significance of asymptomatic bacteriuria in diabetes. Diabetes Care. 2011;34(1):230-235. doi:10.2337/dc10-0421 [PMC free article: PMC3005460] [PubMed: 20937688] [CrossRef]
- 695.
- Ishay A, Lavi I, Luboshitzky R. Prevalence and risk factors for asymptomatic bacteriuria in women with type 2 diabetes mellitus. Diabet Med. 2006;23(2):185-188. doi:10.1111/j.1464-5491.2005.01758.x [PubMed: 16433717] [CrossRef]
- 696.
- Bonadio M, Boldrini E, Forotti G, et al. Asymptomatic bacteriuria in women with diabetes: influence of metabolic control. Clin Infect Dis. 2004;38(6):e41-e45. doi:10.1086/381755 [PubMed: 14999644] [CrossRef]
- 697.
- Makuyana D, Mhlabi D, Chipfupa M, Munyombwe T, Gwanzura L. Asymptomatic bacteriuria among outpatients with diabetes mellitus in an urban black population. Cent Afr J Med. 2002;48(7-8):78-82. doi:10.4314/cajm.v48i7.8433 [PubMed: 14562524] [CrossRef]
- 698.
- Keleştimur F, Unal A, Paşaoğlu H, Başar E, Kiliç H, Doğanay M. [Asymptomatic bacteriuria in patients with diabetes mellitus] [Article in Turkish]. Mikrobiyol Bul. 1990;24(2):126-132. [PubMed: 2089231]
- 699.
- Schmitt JK, Fawcett CJ, Gullickson G. Asymptomatic bacteriuria and hemoglobin A1. Diabetes Care. 1986;9(5):518-520. doi:10.2337/diacare.9.5.518 [PubMed: 3769720] [CrossRef]
- 700.
- Abu-Bakare A, Oyaide SM. Asymptomatic bacteriuria in Nigerian diabetics. J Trop Med Hyg. 1986;89(1):29-32. [PubMed: 3528518]
- 701.
- Rózsai B, Lányi E, Berki T, Soltész G. Urinary cytokine response to asymptomatic bacteriuria in type 1 diabetic children and young adults. Pediatr Diabetes. 2006;7(3):153-158. doi:10.1111/j.1399-543X.2006.00161.x [PubMed: 16787522] [CrossRef]
- 702.
- Mendoza T, García de los Ríos M, Lafourcade M, Soto C, Durruty P, Alvo M. [Asymptomatic bacteriuria in type 2 diabetics women] [Article in Spanish]. Rev Med Chil. 2002;130(9):1001-1007. [PubMed: 12434647]
- 703.
- Vigg B, Rai V. Asymptomatic bacteriuria in diabetics. J Assoc Physicians India. 1977;25(1):57-61. [PubMed: 924956]
- 704.
- Joffe BI, Seftel HC, Distiller LA. Asymptomatic bacteriuria in diabetes mellitus. S Afr Med J. 1974;48(30):1306-1308. [PubMed: 4604134]
- 705.
- Rózsai B, Lányi E, Soltész G. Asymptomatic bacteriuria and leukocyturia in type 1 diabetic children and young adults. Diabetes Care. 2003;26(7):2209-2210. doi:10.2337/diacare.26.7.2209-a [PubMed: 12832338] [CrossRef]
- 706.
- Boroumand MA, Sam L, Abbasi SH, Salarifar M, Kassaian E, Forghani S. Asymptomatic bacteriuria in type 2 Iranian diabetic women: a cross sectional study. BMC Womens Health. 2006;6:4. doi:10.1186/1472-6874-6-4 [PMC free article: PMC1402268] [PubMed: 16504076] [CrossRef]
- 707.
- Zhanel GG, Nicolle LE, Harding GK. Prevalence of asymptomatic bacteriuria and associated host factors in women with diabetes mellitus. The Manitoba Diabetic Urinary Infection Study Group. Clin Infect Dis. 1995;21(2):316-322. doi:10.1093/clinids/21.2.316 [PubMed: 8562738] [CrossRef]
- 708.
- Boyko EJ, Fihn SD, Scholes D, Abraham L, Monsey B. Risk of urinary tract infection and asymptomatic bacteriuria among diabetic and nondiabetic postmenopausal women. Am J Epidemiol. 2005;161(6):557-564. doi:10.1093/aje/kwi078 [PubMed: 15746472] [CrossRef]
- 709.
- Sotiropoulos A, Skourtis S, Merkouris P, et al. Incidence and outcome of asymptomatic bacteriuria in females with type 2 diabetes mellitus over a 1-year follow-up period and association with risk factors. Diabet Med. 2005;22(11):1625-1626. doi:10.1111/j.1464-5491.2005.01664.x [PubMed: 16241934] [CrossRef]
- 710.
- Geerlings SE, Stolk RP, Camps MJ, et al. Asymptomatic bacteriuria may be considered a complication in women with diabetes. Diabetes Mellitus Women Asymptomatic Bacteriuria Utrecht Study Group. Diabetes Care 2000;23(6):744-749. doi:10.2337/diacare.23.6.744 [PubMed: 10840989] [CrossRef]
- 711.
- Geerlings SE, Stolk RP, Camps MJ, et al. Consequences of asymptomatic bacteriuria in women with diabetes mellitus. Arch Intern Med. 2001;161(11):1421-1427. doi:10.1001/archinte.161.11.1421 [PubMed: 11386891] [CrossRef]
- 712.
- Sawers JS, Todd WA, Kellett HA, et al. Bacteriuria and autonomic nerve function in diabetic women. Diabetes Care. 1986;9(5):460-464. doi:10.2337/diacare.9.5.460 [PubMed: 3533474] [CrossRef]
- 713.
- Brauner A, Flodin U, Hylander B, Ostenson CG. Bacteriuria, bacterial virulence and host factors in diabetic patients. Diabet Med. 1993;10(6):550-554. doi:10.1111/j.1464-5491.1993.tb00119.x [PubMed: 8365092] [CrossRef]
- 714.
- Hansen RO. Bacteriuria in diabetic and non-diabetic out-patients. Acta Med Scand. 1964;176:721-730. doi:10.1111/j.0954-6820.1964.tb00680.x [PubMed: 14259911] [CrossRef]
- 715.
- Vejlsgaard R. Studies on urinary infection in diabetics. I. Bacteriuria in patients with diabetes mellitus and in control subjects. Acta Med Scand. 1966;179(2):173-182. [PubMed: 5325464]
- 716.
- Ooi BS, Chen BT, Yu M. Prevalence and site of bacteriuria in diabetes mellitus. Postgrad Med J. 1974;50(586):497-499. doi:10.1136/pgmj.50.586.497 [PMC free article: PMC2495655] [PubMed: 4464512] [CrossRef]
- 717.
- Jaspan JB, Mangera C, Krut LH. Bacteriuria in Black diabetics. S Afr Med J. 1977;51(12):374-376. [PubMed: 847559]
- 718.
- Forland M, Thomas V, Shelokov A. Urinary tract infections in patients with diabetes mellitus. Studies on antibody coating of bacteria. JAMA. 1977;238(18):1924-1926. doi:10.1001/jama.1977.03280190026020 [PubMed: 333137] [CrossRef]
- 719.
- Huvos A, Rocha H. Frequency of bacteriuria in patients with diabetes mellitus: a controlled study. N Engl J Med. 1959;261(24):1213-1216. doi:10.1056/NEJM195912102612403 [PubMed: 14405661] [CrossRef]
- 720.
- O’Sullivan DJ, Fitzgerald MG, Meynell MJ, Malins JM. Urinary tract infection. A comparative study in the diabetic and general populations. Br Med J. 1961;1(5228):786-788. doi:10.1136/bmj.1.5228.786 [PMC free article: PMC1953843] [PubMed: 13730121] [CrossRef]
- 721.
- Karunajeewa H, McGechie D, Stuccio G, Stingemore N, Davis WA, Davis TME. Asymptomatic bacteriuria as a predictor of subsequent hospitalisation with urinary tract infection in diabetic adults: the Fremantle Diabetes Study. Diabetologia. 2005;48(7):1288-1291. doi:10.1007/s00125-005-1794-3 [PubMed: 15918016] [CrossRef]
- 722.
- Harding GK, Zhanel GG, Nicolle LE, Cheang M, Manitoba Diabetes Urinary Tract Infection Study Group. Antimicrobial treatment in diabetic women with asymptomatic bacteriuria. N Engl J Med. 2002;347(20):1576-1583. doi:10.1056/NEJMoa021042 [PubMed: 12432044] [CrossRef]
- 723.
- Ribera MC, Pascual R, Orozco D, Pérez Barba C, Pedrera V, Gil V. Incidence and risk factors associated with urinary tract infection in diabetic patients with and without asymptomatic bacteriuria. Eur J Clin Microbiol Infect Dis. 2006;25(6):389-393. doi:10.1007/s10096-006-0148-5 [PubMed: 16767487] [CrossRef]
- 724.
- Semetkowska-Jurkiewicz E, Horoszek-Maziarz S, Galiński J, Manitius A, Krupa-Wojciechowska B. The clinical course of untreated asymptomatic bacteriuria in diabetic patients—14-year follow-up. Mater Med Pol. 1995;27(3):91-95. [PubMed: 8935144]
- 725.
- Czaja CA, Rutledge BN, Cleary PA, et al. Urinary tract infections in women with type 1 diabetes mellitus: survey of female participants in the Epidemiology of Diabetes Interventions and Complications study cohort. J Urol. 2009;181(3):1129-1134. doi:10.1016/j.juro.2008.11.021 [PMC free article: PMC2699609] [PubMed: 19152925] [CrossRef]
- 726.
- Foy MC, Estrella MM, Lucas GM, et al. Comparison of risk factors and outcomes in HIV immune complex kidney disease and HIV-associated nephropathy. Clin J Am Soc Nephrol. 2013;8(9):1524-1532. doi:10.2215/CJN.10991012 [PMC free article: PMC3805081] [PubMed: 23685946] [CrossRef]
- 727.
- Flandre P, Pugliese P, Cuzin L, et al. Risk factors of chronic kidney disease in HIV-infected patients. Clin J Am Soc Nephrol. 2011;6(7):1700-1707. doi:10.2215/cjn.09191010 [PubMed: 21566114] [CrossRef]
- 728.
- Fernando SK, Finkelstein FO, Moore BA, Weissman S. Prevalence of chronic kidney disease in an urban HIV infected population. Am J Med Sci. 2008;335(2):89-94. doi:10.1097/MAJ.0b013e31812e6b34 [PubMed: 18277114] [CrossRef]
- 729.
- Nasr SH, Markowitz GS, Stokes MB, Said SM, Valeri AM, D’Agati VD. Acute postinfectious glomerulonephritis in the modern era: experience with 86 adults and review of the literature. Medicine (Baltimore). 2008;87(1):21-32. doi:10.1097/md.0b013e318161b0fc [PubMed: 18204367] [CrossRef]
- 730.
- Kim PS, Woods C, Dutcher L, et al. Increased prevalence of albuminuria in HIV-infected adults with diabetes. PLoS One. 2011;6(9):e24610. doi:10.1371/journal.pone.0024610 [PMC free article: PMC3172239] [PubMed: 21931772] [CrossRef]
- 731.
- Zaidan M, Lescure FX, Brochériou I, et al. Tubulointerstitial nephropathies in HIV-infected patients over the past 15 years: a clinico-pathological study. Clin J Am Soc Nephrol. 2013;8(6):930-938. doi:10.2215/CJN.10051012 [PMC free article: PMC3675854] [PubMed: 23430209] [CrossRef]
- 732.
- United States Renal Data System. 2023 USRDS Annual Data Report: Epidemiology of Kidney Disease in the United States. National Institutes of Health, National Institute of Diabetes and Digestive and Kidney Diseases. Accessed November 22, 2023. https://usrds-adr
.niddk.nih.gov/2023 - 733.
- Geetha D, Kronbichler A, Rutter M, et al. Impact of the COVID-19 pandemic on the kidney community: lessons learned and future directions. Nat Rev Nephrol. 2022;18(11):724-737. doi:10.1038/s41581-022-00618-4 [PMC free article: PMC9400561] [PubMed: 36002770] [CrossRef]
- 734.
- Yang Y, Zou S, Xu G. An update on the interaction between COVID-19, vaccines, and diabetic kidney disease. Front Immunol. 2022;13:999534. doi:10.3389/fimmu.2022.999534 [PMC free article: PMC9630353] [PubMed: 36341356] [CrossRef]
- 735.
- Qi Z, Yuan S, Wei J, et al. Clinical and pathological features of omicron variant of SARS-CoV-2-associated kidney injury. J Med Virol. 2023;95(10):e29196. doi:10.1002/jmv.29196 [PubMed: 37881096] [CrossRef]
- 736.
- Wu H, Larsen CP, Hernandez-Arroyo CF, et al. AKI and collapsing glomerulopathy associated with COVID-19 and APOL 1 high-risk genotype. J Am Soc Nephrol. 2020;31(8):1688-1695. doi:10.1681/ASN.2020050558 [PMC free article: PMC7460910] [PubMed: 32561682] [CrossRef]
- 737.
- May RM, Cassol C, Hannoudi A, et al. A multi-center retrospective cohort study defines the spectrum of kidney pathology in coronavirus 2019 disease (COVID-19). Kidney Int. 2021;100(6):1303-1315. doi:10.1016/j.kint.2021.07.015 [PMC free article: PMC8328528] [PubMed: 34352311] [CrossRef]
- 738.
- Scirica BM, Bhatt DL, Braunwald E, et al. Saxagliptin and cardiovascular outcomes in patients with type 2 diabetes mellitus. N Engl J Med. 2013;369(14):1317-1326. doi:10.1056/NEJMoa1307684 [PubMed: 23992601] [CrossRef]
- 739.
- Rosenstock J, Perkovic V, Johansen OE, et al. Effect of linagliptin vs placebo on major Cardiovascular events in adults with type 2 diabetes and high cardiovascular and renal risk: the CARMELINA randomized clinical trial. JAMA. 2019;321(1):69-79. doi:10.1001/jama.2018.18269 [PMC free article: PMC6583576] [PubMed: 30418475] [CrossRef]
- 740.
- Rosenstock J, Perkovic V, Alexander JH, et al. Rationale, design, and baseline characteristics of the CArdiovascular safety and Renal Microvascular outcomE study with LINAgliptin (CARMELINA®): a randomized, double-blind, placebo-controlled clinical trial in patients with type 2 diabetes and high cardio-renal risk. Cardiovasc Diabetol. 2018;17(1):39. doi:10.1186/s12933-018-0682-3 [PMC free article: PMC5870815] [PubMed: 29540217] [CrossRef]
Drs. Pavkov, Koyama, and Nelson reported no conflicts of interest. Dr. Bjornstad reported serving or having served as a consultant for AstraZeneca, Bayer, Bristol-Myers Squibb, Boehringer Ingelheim, Eli Lilly, LG Chemistry, Sanofi, Novo Nordisk, and Horizon Pharma; he also serves or has served on the advisory boards and/or steering committees of AstraZeneca, Bayer, Boehringer Ingelheim, Novo Nordisk, and XORTX.
- Summary
- Introduction
- Sources and Limitations of National Data on Kidney Disease and Diabetes
- Definition, Measurements, and Classification
- Pathophysiology and Clinical Course
- Elevated Albuminuria
- Kidney Failure
- Risk Factors for Diabetes-Related Kidney Disease
- Treatment of Diabetes-Related Kidney Disease
- Other Kidney Diseases Associated With Infections in Persons With Diabetes
- Conclusions
- List of Abbreviations
- Conversions
- Acknowledgment
- Article History
- Disclaimer
- References
- Kidney Disease and Diabetes - Diabetes in AmericaKidney Disease and Diabetes - Diabetes in America
Your browsing activity is empty.
Activity recording is turned off.
See more...